Insights into Short Chain Fatty Acids and Gut Microbiota
Discover how Short Chain Fatty Acids and Gut Microbiota influence health, with insights into dietary fiber, genetics, and gut wellness. Learn more now.
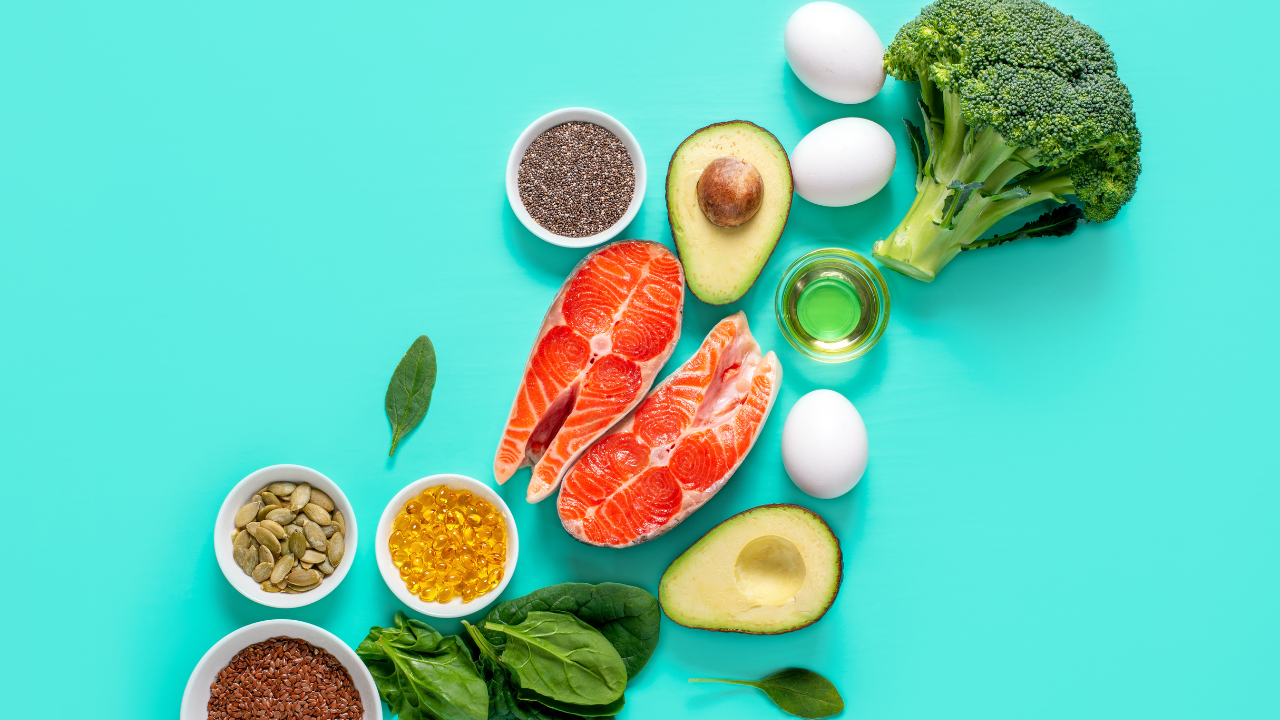
The interplay of short-chain fatty acids and the microbiota in the gut is of great importance in the field of personalized nutrition and gut health.
- Short chain fatty acids (SCFAs) are important for our digestive system.
- SCFAs come from dietary fiber.
- They have various roles in maintaining health.
- They influence microbiome diversity.
- They affect how our body responds to food.
Main Findings
- Short-chain fatty acids (SCFAs) are crucial for gut health, produced by the microbiota from dietary fiber.
- SCFA production and metabolism vary significantly between individuals due to genetic and environmental factors.
- The relationship between dietary fiber, SCFAs, and gut microbiota influences various health outcomes.
- Different gut microbiota compositions, or enterotypes, affect the body's response to dietary fiber and SCFA production.
- Genetic variations (genotypes) influence how the gut microbiota interacts with dietary components and metabolizes SCFAs.
- SCFAs play a role in immune regulation, with implications for chronic diseases like obesity, diabetes, and heart disease.
The Basics of Microbiota and SCFAs
The Impact of Short-Chain Fatty Acids on Human Health
In the intricate world of our bodies, there's a hidden community of microbes known as the microbiota, including bacteria, fungi, and more ✅ Trusted Source
➤ Go to source
Sender, R.; Fuchs, S.; Milo, R. Revised Estimates for the Number of Human and Bacteria Cells in the Body. PLoS Biol. 2016, 14, e1002533.
➤ Get "Smart Citations"
(hover to see more details)
.
This microbiota, residing mainly in our colon, collaborates with us in ways we're just beginning to understand, akin to an ancient partnership.
This fascinating synergy is the foundation of the holobiont theory ✅ Trusted Source
➤ Go to source
Zilber-Rosenberg, I.; Rosenberg, E. Role of microorganisms in the evolution of animals and plants: The hologenome theory of evolution. FEMS Microbiol. Rev. 2008, 32, 723–735.
➤ Get "Smart Citations"
(hover to see more details)
.
Recent research has unveiled the microbiota's pivotal role in regulating metabolic, immune, and endocrine functions, while also shaping our response to diseases ✅ Trusted Source
➤ Go to source
Sommer, F.; Bäckhed, F. The gut microbiota—Masters of host development and physiology. Nat. Rev. Microbiol. 2013, 11, 227–238.
➤ Get "Smart Citations"
(hover to see more details)
.
Imbalances in the gut microbiota have been linked to various chronic health issues, including obesity ✅ Trusted Source
➤ Go to source
Perry, R.J.; Peng, L.; Barry, N.A.; Cline, G.W.; Zhang, D.; Cardone, R.L.; Petersen, K.F.; Kibbey, R.G.; Goodman, A.L.; Shulman, G.I. Acetate mediates a microbiome-brain-β cell axis promoting metabolic syndrome. Nature 2016, 7606, 213–217.
➤ Get "Smart Citations"
(hover to see more details)
, cardiovascular disease ✅ Trusted Source
➤ Go to source
McRae, M.P. Dietary Fiber is Beneficial for the Prevention of Cardiovascular Disease: An Umbrella Review of Meta-analyses. J. Chiropr. Med. 2017, 16, 289–299.
➤ Get "Smart Citations"
(hover to see more details)
, diabetes ✅ Trusted Source
➤ Go to source
Perry, R.J.; Peng, L.; Barry, N.A.; Cline, G.W.; Zhang, D.; Cardone, R.L.; Petersen, K.F.; Kibbey, R.G.; Goodman, A.L.; Shulman, G.I. Acetate mediates a microbiome-brain-β cell axis promoting metabolic syndrome. Nature 2016, 7606, 213–217.
➤ Get "Smart Citations"
(hover to see more details)
✅ Trusted Source
➤ Go to source
Akshintala, V.S.; Talukdar, R.; Singh, V.K.; Goggins, M. The Gut Microbiome in Pancreatic Disease. Clin. Gastroenterol. Hepatol. 2018, 17, 290–295.
➤ Get "Smart Citations"
(hover to see more details)
, autoimmune disorders ✅ Trusted Source
➤ Go to source
Diamanti, A.P.; Rosado, M.M.; Laganà, B.; D’Amelio, R. Microbiota and chronic inflammatory arthritis: An interwoven link. J. Transl. Med. 2016, 14, 233.
➤ Get "Smart Citations"
(hover to see more details)
✅ Trusted Source
➤ Go to source
Kang, L.; Li, P.; Wang, D.; Wang, T.; Hao, D.; Qu, X. Alterations in intestinal microbiota diversity, composition, and function in patients with sarcopenia. Nature 2021, 11, 4628.
➤ Get "Smart Citations"
(hover to see more details)
, and even neurodegenerative diseases ✅ Trusted Source
➤ Go to source
Gentile, F.; Doneddu, P.E.; Riva, N.; Nobile-Orazio, E.; Quattrini, A. Diet, Microbiota and Brain Health: Unraveling the Network Intersecting Metabolism and Neurodegeneration. Int. J. Mol. Sci. 2020, 21, 7471.
➤ Get "Smart Citations"
(hover to see more details)
✅ Trusted Source
➤ Go to source
Friedland, R.P. Mechanisms of Molecular Mimicry Involving the Microbiota in Neurodegeneration. J. Alzheimer’s Dis. 2015, 45, 349–352.
➤ Get "Smart Citations"
(hover to see more details)
like Parkinson's ✅ Trusted Source
➤ Go to source
Baldini, F.; Hertel, J.; Sandt, E.; Thinnes, C.C.; Neuberger-Castillo, L.; Pavelka, L.; Betsou, F.; Krüger, R.; Thiele, I. Parkinson’s disease-associated alterations of the gut microbiome predict disease relevant changes in metabolic functions. BMC Biol. 2020, 18, 62.
➤ Get "Smart Citations"
(hover to see more details)
and Alzheimer's ✅ Trusted Source
➤ Go to source
Goyal, D.; Ali, S.A.; Singh, R.K. Emerging role of gut microbiota in modulation of neuroinflammation and neurodegeneration with emphasis on Alzheimer’s disease. Prog. Neuropsychopharmacol. Biol. Psychiatry 2021, 106, 9.
➤ Get "Smart Citations"
(hover to see more details)
.
Dietary habits have emerged as a powerful influencer of our gut health and overall well-being ✅ Trusted Source
➤ Go to source
McRae, M.P. Dietary Fiber is Beneficial for the Prevention of Cardiovascular Disease: An Umbrella Review of Meta-analyses. J. Chiropr. Med. 2017, 16, 289–299.
➤ Get "Smart Citations"
(hover to see more details)
✅ Trusted Source
➤ Go to source
Pan, A.; Lin, X.; Hemler, E.; Hu, F.B. Diet and Cardiovascular Disease: Advances and Challenges in Population-Based Studies. Cell Metab. 2018, 27, 489–496.
➤ Get "Smart Citations"
(hover to see more details)
✅ Trusted Source
➤ Go to source
Christ, A.; Latz, E. The Western lifestyle has lasting effects on metaflammation. Nat. Rev. Immunol. 2019, 19, 267–268.
➤ Get "Smart Citations"
(hover to see more details)
✅ Trusted Source
➤ Go to source
Devore, E.E.; Kang, J.H.; Breteler, M.M.B.; Grodstein, F. Dietary intake of berries and flavonoids in relation to cognitive decline. Ann. Neurol. 2012, 72, 135–143.
➤ Get "Smart Citations"
(hover to see more details)
✅ Trusted Source
➤ Go to source
Darmadi-Blackberry, I.; Wahlqvist, M.L.; Kouris-Blazos, A.; Steen, B.; Lukito, W.; Horie, Y.; Horie, K. Legumes: The most important dietary predictor of survival in older people of different ethnicities. Asia Pac. J. Clin. Nutr. 2004, 13, 217–220. ✅ Trusted Source
➤ Go to source
Katagiri, R.; Goto, A.; Sawada, N.; Yamaji, T.; Iwasaki, M.; Noda, M.; Iso, H.; Tsugane, S. Dietary fiber intake and total and cause-specific mortality: The Japan Public Health Center-based prospective study. Am. J. Clin. Nutr. 2020, 111, 1027–1035.
➤ Get "Smart Citations"
(hover to see more details)
✅ Trusted Source
➤ Go to source
Szic, K.S.V.; Declerck, K.; Vidaković, M.; Berghe, W.V. From inflammaging to healthy aging by dietary lifestyle choices: Is epigenetics the key to personalized nutrition? Clin. Epigenetics 2015, 7, 33.
➤ Get "Smart Citations"
(hover to see more details)
✅ Trusted Source
➤ Go to source
Fitzgerald, K.C.; Tyry, T.; Salter, A.; Cofield, S.S.; Cutter, G.; Fox, R.; Marrie, R.A. Diet quality is associated with disability and symptom severity in multiple sclerosis. Neurology 2018, 90, e1–e11.
➤ Get "Smart Citations"
(hover to see more details)
.
The decline in dietary fiber (DF) consumption, for example, has raised concerns.
DF, found in foods like fruits, vegetables, and grains, serves as a bridge between our gut microbiota and our health ✅ Trusted Source
➤ Go to source
Gill, S.K.; Rossi, M.; Bajka, B.; Whelan, K. Dietary fibre in gastrointestinal health and disease. Nat. Rev. Gastroenterol. Hepatol. 2021, 18, 101–116.
➤ Get "Smart Citations"
(hover to see more details)
✅ Trusted Source
➤ Go to source
Dingeo, G.; Brito, A.; Samouda, H.; Iddir, M.; Frano, M.R.L.; Bohn, T. Phytochemicals as modifiers of gut microbial communities. Food Funct. 2020, 11, 8444–8471.
➤ Get "Smart Citations"
(hover to see more details)
✅ Trusted Source
➤ Go to source
Makki, K.; Deehan, E.C.; Walter, J.; Bäckhed, F. The Impact of Dietary Fiber on Gut Microbiota in Host Health and Disease. Cell Host Microbe 2018, 23, 705–716.
➤ Get "Smart Citations"
(hover to see more details)
✅ Trusted Source
➤ Go to source
Jha, S.K.; Singh, H.R.; Prakash, P. Dietary Fiber and Human Health: An Introduction; Elsevier: Amsterdam, The Netherlands, 2017; pp. 1–22.
➤ Get "Smart Citations"
(hover to see more details)
✅ Trusted Source
➤ Go to source
Desai, M.S.; Seekatz, A.M.; Koropatkin, N.M.; Kamada, N.; Hickey, C.A.; Wolter, M.; Pudlo, N.A.; Kitamoto, S.; Terrapon, N.; Muller, A.; et al. A Dietary Fiber-Deprived Gut Microbiota Degrades the Colonic Mucus Barrier and Enhances Pathogen Susceptibility. Cell 2016, 167, 1339–1353.
➤ Get "Smart Citations"
(hover to see more details)
✅ Trusted Source
➤ Go to source
Tan, J.; McKenzie, C.; Vuillermin, P.J.; Goverse, G.; Vinuesa, C.G.; Mebius, R.E.; Macia, L.; Mackay, C.R. Dietary Fiber and Bacterial SCFA Enhance Oral Tolerance and Protect against Food Allergy through Diverse Cellular Pathways. Cell Rep. 2016, 15, 2809–2824.
➤ Get "Smart Citations"
(hover to see more details)
✅ Trusted Source
➤ Go to source
Kuo, S.-M. The interplay between fiber and the intestinal microbiome in the inflammatory response. Adv. Nutr. 2013, 4, 16–28.
➤ Get "Smart Citations"
(hover to see more details)
.
It's a unique substance that our digestive enzymes can't fully break down but can be utilized by our gut microbes.
They turn DF into short-chain fatty acids (SCFA), such as butyrate, acetate, and propionate ✅ Trusted Source
➤ Go to source
Dingeo, G.; Brito, A.; Samouda, H.; Iddir, M.; Frano, M.R.L.; Bohn, T. Phytochemicals as modifiers of gut microbial communities. Food Funct. 2020, 11, 8444–8471.
➤ Get "Smart Citations"
(hover to see more details)
.
SCFA, particularly butyrate, plays a crucial role in nourishing the cells lining our colon and supporting our immune system ✅ Trusted Source
➤ Go to source
Gill, P.A.; Zelm, M.C.V.; Muir, J.G.; Gibson, P.R. Review article: Short chain fatty acids as potential therapeutic agents in human gastrointestinal and inflammatory disorders. Aliment. Pharmacol. Ther. 2018, 48, 15–34.
➤ Get "Smart Citations"
(hover to see more details)
✅ Trusted Source
➤ Go to source
Louis, P.; Hold, G.L.; Flint, H.J. The gut microbiota, bacterial metabolites and colorectal cancer. Nat. Rev. Microbiol. 2014, 12, 661–672.
➤ Get "Smart Citations"
(hover to see more details)
✅ Trusted Source
➤ Go to source
Singh, N.; Gurav, A.; Sivaprakasam, S.; Brady, E.; Padia, R.; Shi, H.; Thangaraju, M.; Prasad, P.D.; Manicassamy, S.; Munn, D.H.; et al. Activation of Gpr109a, Receptor for Niacin and the Commensal Metabolite Butyrate, Suppresses Colonic Inflammation and Carcinogenesis. Immunity 2014, 40, 128–139.
➤ Get "Smart Citations"
(hover to see more details)
✅ Trusted Source
➤ Go to source
Chang, P.V.; Hao, L.; Offermanns, S.; Medzhitov, R. The microbial metabolite butyrate regulates intestinal macrophage function via histone deacetylase inhibition. Proc. Natl. Acad. Sci. USA 2014, 111, 2247–2252.
➤ Get "Smart Citations"
(hover to see more details)
✅ Trusted Source
➤ Go to source
Furusawa, Y.; Obata, Y.; Fukuda, S.; Endo, T.A.; Nakato, G.; Takahashi, D.; Nakanishi, Y.; Uetake, C.; Kato, K.; Kato, T.; et al. Commensal microbe-derived butyrate induces the differentiation of colonic regulatory T cells. Nature 2013, 504, 446–450.
➤ Get "Smart Citations"
(hover to see more details)
✅ Trusted Source
➤ Go to source
Hamer, H.M.; Jonkers, D.; Venema, K.; Vanhoutvin, S.; Troost, F.J.; Brummer, R.J. Review article: The role of butyrate on colonic function. Aliment. Pharmacol. Ther. 2007, 27, 104–119.
➤ Get "Smart Citations"
(hover to see more details)
.
Additionally, SCFA act as powerful immune regulators, affecting different tissues and systems throughout our body ✅ Trusted Source
➤ Go to source
Gill, S.K.; Rossi, M.; Bajka, B.; Whelan, K. Dietary fibre in gastrointestinal health and disease. Nat. Rev. Gastroenterol. Hepatol. 2021, 18, 101–116.
➤ Get "Smart Citations"
(hover to see more details)
✅ Trusted Source
➤ Go to source
Gill, P.A.; Zelm, M.C.V.; Muir, J.G.; Gibson, P.R. Review article: Short chain fatty acids as potential therapeutic agents in human gastrointestinal and inflammatory disorders. Aliment. Pharmacol. Ther. 2018, 48, 15–34.
➤ Get "Smart Citations"
(hover to see more details)
✅ Trusted Source
➤ Go to source
Reichardt, N.; Vollmer, M.; Holtrop, G.; Farquharson, F.M.; Wefers, D.; Bunzel, M.; Duncan, S.H.; Drew, J.E.; Williams, L.M.; Milligan, G.; et al. Specific substrate-driven changes in human faecal microbiota composition contrast with functional redundancy in short-chain fatty acid production. ISME J. 2018, 12, 610–622.
➤ Get "Smart Citations"
(hover to see more details)
.
The importance of SCFA in maintaining our health cannot be overstated ✅ Trusted Source
➤ Go to source
Durack, J.; Christophersen, C.T. Human Respiratory and Gut Microbiomes—Do They Really Contribute to Respiratory Health? Front. Pediatrics 2020, 8, 528.
➤ Get "Smart Citations"
(hover to see more details)
✅ Trusted Source
➤ Go to source
Levan, S.R.; Stamnes, K.A.; Lin, D.L.; Panzer, A.R.; Fukui, E.; McCauley, K.; Fujimura, K.E.; McKean, M.; Ownby, D.R.; Zoratti, E.M.; et al. Elevated faecal 12,13-diHOME concentration in neonates at high risk for asthma is produced by gut bacteria and impedes immune tolerance. Nat. Microbiol. 2019, 4, 1851–1861.
➤ Get "Smart Citations"
(hover to see more details)
✅ Trusted Source
➤ Go to source
Durack, J.; Kimes, N.E.; Lin, D.L.; Rauch, M.; McKean, M.; McCauley, K.; Panzer, A.R.; Mar, J.S.; Cabana, M.D.; Lynch, S.V. Delayed gut microbiota development in high-risk for asthma infants is temporarily modifiable by Lactobacillus supplementation. Nat. Commun. 2018, 9, 707.
➤ Get "Smart Citations"
(hover to see more details)
✅ Trusted Source
➤ Go to source
Fujimura, K.E.; Sitarik, A.R.; Havstad, S.; Lin, D.L.; Levan, S.; Fadrosh, D.; Panzer, A.R.; LaMere, B.; Rackaityte, E.; Lukacs, N.W.; et al. Neonatal gut microbiota associates with childhood multi– sensitized atopy and T–cell differentiation. Nat. Med. 2016, 22, 1187–1191.
➤ Get "Smart Citations"
(hover to see more details)
✅ Trusted Source
➤ Go to source
Maslowski, K.M.; Vieira, A.T.; Ng, A.; Kranich, J.; Sierro, F.; Di, Y.; Schilter, H.C.; Rolph, M.S.; Mackay, F.; Artis, D.; et al. Regulation of inflammatory responses by gut microbiota and chemoattractant receptor GPR43. Nature 2009, 461, 1282–1286.
➤ Get "Smart Citations"
(hover to see more details)
✅ Trusted Source
➤ Go to source
Blacher, E.; Levy, M.; Tatirovsky, E.; Elinav, E. Microbiome-Modulated Metabolites at the Interface of Host Immunity. J. Immunol. 2017, 198, 572–580.
➤ Get "Smart Citations"
(hover to see more details)
.
But it's not just our diet that influences our gut microbiota.
Various factors, including genetics, age, medication, and exposure to pathogens, also play significant roles ✅ Trusted Source
➤ Go to source
Dingeo, G.; Brito, A.; Samouda, H.; Iddir, M.; Frano, M.R.L.; Bohn, T. Phytochemicals as modifiers of gut microbial communities. Food Funct. 2020, 11, 8444–8471.
➤ Get "Smart Citations"
(hover to see more details)
✅ Trusted Source
➤ Go to source
Filippis, F.D.; Pellegrini, N.; Vannini, L.; Jeffery, I.B.; Storia, A.L.; Laghi, L.; Serrazanetti, D.I.; Cagno, R.D.; Ferrocino, I.; Lazzi, C.; et al. High-level adherence to a Mediterranean diet beneficially impacts the gut microbiota and associated metabolome. BMJ Gut 2016, 65, 1812–1821.
➤ Get "Smart Citations"
(hover to see more details)
✅ Trusted Source
➤ Go to source
Martínez, G.P.; Bäuerl, C.; Collado, M.C. Understanding gut microbiota in elderly’s health will enable intervention through probiotics. Benef. Microbes 2014, 3, 235–246.
➤ Get "Smart Citations"
(hover to see more details)
✅ Trusted Source
➤ Go to source
Wu, G.D.; Chen, J.; Hoffmann, C.; Bittinger, K.; Chen, Y.-Y.; Keilbaugh, S.A.; Bewtra, M.; Knights, D.; Walters, W.A.; Knight, R.; et al. Linking Long-Term Dietary Patterns with Gut Microbial Enterotypes. Science 2011, 334, 105–109.
➤ Get "Smart Citations"
(hover to see more details)
✅ Trusted Source
➤ Go to source
Markiewicz, L.H.; Honke, J.; Haros, M.; Swiaztecka, D.; Wróblewska, B. Diet shapes the ability of human intestinal microbiota to degrade phytate—In vitro studies. J. Appl. Microbiol. 2013, 115, 247–259.
➤ Get "Smart Citations"
(hover to see more details)
✅ Trusted Source
➤ Go to source
David, L.A.; Maurice, C.F.; Carmody, R.N.; Gootenberg, D.B.; Button, J.E.; Wolfe, B.E.; Ling, A.V.; Devlin, A.S.; Varma, Y.; Fischbach, M.A.; et al. Diet rapidly and reproducibly alters the human gut microbiome. Nature 2014, 505, 559–563.
➤ Get "Smart Citations"
(hover to see more details)
.
Genetic variations, like single nucleotide polymorphisms (SNPs), can impact how our body responds to DF intake and SCFA production ✅ Trusted Source
➤ Go to source
Goodrich, J.K.; Davenport, E.R.; Clark, A.G.; Ley, R.E. The Relationship Between the Human Genome and Microbiome Comes into View. Annu. Rev. Genet. 2017, 51, 413–433.
➤ Get "Smart Citations"
(hover to see more details)
✅ Trusted Source
➤ Go to source
Singh, N.; Gurav, A.; Sivaprakasam, S.; Brady, E.; Padia, R.; Shi, H.; Thangaraju, M.; Prasad, P.D.; Manicassamy, S.; Munn, D.H.; et al. Activation of Gpr109a, Receptor for Niacin and the Commensal Metabolite Butyrate, Suppresses Colonic Inflammation and Carcinogenesis. Immunity 2014, 40, 128–139.
➤ Get "Smart Citations"
(hover to see more details)
✅ Trusted Source
➤ Go to source
Maslowski, K.M.; Vieira, A.T.; Ng, A.; Kranich, J.; Sierro, F.; Di, Y.; Schilter, H.C.; Rolph, M.S.; Mackay, F.; Artis, D.; et al. Regulation of inflammatory responses by gut microbiota and chemoattractant receptor GPR43. Nature 2009, 461, 1282–1286.
➤ Get "Smart Citations"
(hover to see more details)
✅ Trusted Source
➤ Go to source
Nøhr, M.K.; Egerod, K.L.; Christiansen, S.H.; Gille, A.; Offermanns, S.; Schwartz, T.W.; Møller, M. Expression of the short chain fatty acid receptor GPR41/FFAR3 in autonomic and somatic sensory ganglia. Neuroscience 2015, 290, 126–137.
➤ Get "Smart Citations"
(hover to see more details)
✅ Trusted Source
➤ Go to source
Poul, E.L.; Loison, C.; Struyf, S.; Springael, J.-Y.; Lannoy, V.; Decobecq, M.-E.; Brezillon, S.; Dupriez, V.; Vassart, G.; Damme, J.V.; et al. Functional Characterization of Human Receptors for Short Chain Fatty Acids and Their Role in Polymorphonuclear Cell Activation. J. Biol. Chem. 2003, 278, 25481–25489.
➤ Get "Smart Citations"
(hover to see more details)
.
Furthermore, genes associated with SCFA receptors and transporters, as well as genes involved in mucus production and antioxidant defense mechanisms, can have far-reaching effects on our health outcomes ✅ Trusted Source
➤ Go to source
Hudson, B.D.; Murdoch, H.; Milligan, G. Minireview: The Effects of Species Ortholog and SNP Variation on Receptors for Free Fatty Acids. Mol. Endocrinol. 2013, 27, 1177–1187.
➤ Get "Smart Citations"
(hover to see more details)
✅ Trusted Source
➤ Go to source
Van der Hee, B.; Wells, J.M. Microbial Regulation of Host Physiology by Short-chain Fatty Acids. Trends Microbiol. 2021, 29, 700–712.
➤ Get "Smart Citations"
(hover to see more details)
.
Short Chain Fatty Acids and Gut Microbiota
Understanding Dietary Fiber (DF)
DF refers to the indigestible carbohydrates found in foods like fruits, vegetables, grains, and legumes ✅ Trusted Source
➤ Go to source
Stephen, A.M.; Champ, M.M.-J.; Cloran, S.J.; Fleith, M.; Lieshout, L.V.; Mejborn, H.; Burley, V.J. Dietary fibre in Europe: Current state of knowledge on definitions, sources, recommendations, intakes and relationships to health. Nutr. Res. Rev. 2017, 30, 149–190.
➤ Get "Smart Citations"
(hover to see more details)
✅ Trusted Source
➤ Go to source
EFSA Panel on Dietetic Products, Nutrition and Allergies. Scientific Opinion on Dietary Reference Values for carbohydrates and dietary fibre. EFSA J. 2010, 8, 1462.
➤ Get "Smart Citations"
(hover to see more details)
.
It's the stuff that passes through our digestive system relatively unchanged.
The recommended daily intake for DF varies but generally falls between 25-35 grams for adults, depending on the country and dietary guidelines ✅ Trusted Source
➤ Go to source
Stephen, A.M.; Champ, M.M.-J.; Cloran, S.J.; Fleith, M.; Lieshout, L.V.; Mejborn, H.; Burley, V.J. Dietary fibre in Europe: Current state of knowledge on definitions, sources, recommendations, intakes and relationships to health. Nutr. Res. Rev. 2017, 30, 149–190.
➤ Get "Smart Citations"
(hover to see more details)
.
Some health-conscious voices, like the Physicians Committee for Responsible Medicine (PCRM), even advocate for a higher intake of around 40 grams per day ✅ Trusted Source
➤ Go to source
Medicine, P.C.F.R. Dietary Fibre Recommendations. Available online: https://www.pcrm.org/good-nutrition/nutrition-information/fiber (accessed on 22 October 2021)..
DF plays a critical role in our digestive health.
It's associated with improved bowel function, including regularity and reduced constipation ✅ Trusted Source
➤ Go to source
EFSA Panel on Dietetic Products, Nutrition and Allergies. Scientific Opinion on Dietary Reference Values for carbohydrates and dietary fibre. EFSA J. 2010, 8, 1462.
➤ Get "Smart Citations"
(hover to see more details)
.
More importantly, it's linked to a lower risk of non-communicable diseases (NCDs), such as cardiovascular disease, type 2 diabetes, and colorectal cancer ✅ Trusted Source
➤ Go to source
Reynolds, A.; Mann, J.; Cummings, J.; Winter, N.; Mete, E.; Te Morenga, L. Carbohydrate quality and human health: A series of systematic reviews and meta-analyses. Lancet 2019, 393, 434–445.
➤ Get "Smart Citations"
(hover to see more details)
.
DF and Precision Nutrition
Interestingly, where you get your DF matters.
The source of DF in your diet influences the composition of your gut microbiota.
For instance, a low-fat diet and a low-carbohydrate diet can lead to different types of DF intake ✅ Trusted Source
➤ Go to source
Offringa, L.C.; Hartle, J.C.; Rigdon, J.; Gardner, C.D. Changes in Quantity and Sources of Dietary Fiber from Adopting Healthy Low-Fat vs. Healthy Low-Carb Weight Loss Diets: Secondary Analysis of DIETFITS Weight Loss Diet Study. Nutrients 2021, 13, 3625.
➤ Get "Smart Citations"
(hover to see more details)
.
The former may emphasize whole grains and fruits, while the latter might rely more on vegetables and plant protein sources.
These dietary adaptations can impact your gut microbiota and potentially shape your health outcomes.
Defining Dietary Fiber
DF is a diverse group of compounds, but there's consensus on its definition ✅ Trusted Source
➤ Go to source
Mccleary, B.V. Total Dietary Fiber (CODEX Definition) in Foods and Food Ingredients by a Rapid Enzymatic-Gravimetric Method and Liquid Chromatography: Collaborative Study, First Action 2017.16. J. AOAC Int. 2019, 102, 196–207.
➤ Get "Smart Citations"
(hover to see more details)
✅ Trusted Source
➤ Go to source
Codex Alimentarius Commission. Report of the 30th Session of the Codex Committee on Nutrition and Foods for Special Dietary Uses; No. ALINORM 02/32/26; FAO: Rome, Italy; WHO: Geneva, Switzerland, 2009. .
It includes carbohydrate polymers with three or more monomeric units that human enzymes can't digest.
This definition encompasses various types of DF, from insoluble fibers that add bulk to the stool to soluble fibers that serve as fermentation substrates for our gut microbes.
Beyond Carbohydrates
Interestingly, DF isn't limited to carbohydrates alone.
It can also include non-carbohydrate compounds like lignin and polyphenols.
These compounds, along with resistant proteins, can be substrates for beneficial gut bacteria, leading to the production of metabolites like SCFA.
The Complex World of Fiber Fermentation
Fiber fermentation is a complex process influenced by the chemical and physical structure of DF, as well as the makeup of our gut microbiota.
Some DF types, like oligosaccharides, resistant starches, and pectins, are more likely to contribute to SCFA production.
Others, like lignin and cellulose, tend to pass through our system largely unchanged.
Recent research has spotlighted the significance of Short Chain Fatty Acids (SCFA) in the context of dietary fiber (DF), gut microbiota (GM), and probiotics.
SCFA serve as essential messengers, bridging the communication between the gut microbiota and the host.
This article dives into the multifaceted implications of SCFA metabolism for human health, while staying true to the original citation style ✅ Trusted Source
➤ Go to source
Sakata, T. Pitfalls in short-chain fatty acid research: A methodological review. Anim. Sci. J. 2019, 90, 3–13.
➤ Get "Smart Citations"
(hover to see more details)
.
SCFA: The Communicators Between GM and Host
SCFA, characterized as volatile fatty acids with fewer than six carbon atoms, can take straight or branched-chain forms.
The primary SCFA—acetate (C2), propionate (C3), and butyrate (C4)—contribute to the majority (90–95%) of total SCFA produced by the gut microbiota, primarily through carbohydrate fermentation ✅ Trusted Source
➤ Go to source
Macfarlane, G.T.; Macfarlane, S. Bacteria, Colonic Fermentation, and Gastrointestinal Health. J. AOAC Int. 2012, 95, 50–60.
➤ Get "Smart Citations"
(hover to see more details)
✅ Trusted Source
➤ Go to source
Cummings, J.H.; Gibson, G.R.; Macfarlane, G.T. Quantitative estimates of fermentation in the hind gut of man. Acta Vet. Scand. Suppl. 1989, 86, 76–82. .
Recently, it has been unveiled that caproate and valerate, once considered dietary components, also stem from the gut microbiota and are notably increased in individuals with severe obesity ✅ Trusted Source
➤ Go to source
Rios-Covian, D.; González, S.; Nogacka, A.M.; Arboleya, S.; Salazar, N.; Gueimonde, M.; de Los Reyes-Gavilán, C.G. An Overview on Fecal Branched Short-Chain Fatty Acids Along Human Life and as Related with Body Mass Index: Associated Dietary and Anthropometric Factors. Front. Microbiol. 2020, 11, 973.
➤ Get "Smart Citations"
(hover to see more details)
.
Branched-chain SCFA (BCFA), including isobutyrate, isovalerate, and 2-methylbutanoate, make up a smaller portion (up to 5%) of total SCFA production, originating from the metabolism of specific amino acids ✅ Trusted Source
➤ Go to source
Macfarlane, G.T.; Macfarlane, S. Bacteria, Colonic Fermentation, and Gastrointestinal Health. J. AOAC Int. 2012, 95, 50–60.
➤ Get "Smart Citations"
(hover to see more details)
✅ Trusted Source
➤ Go to source
Rios-Covian, D.; González, S.; Nogacka, A.M.; Arboleya, S.; Salazar, N.; Gueimonde, M.; de Los Reyes-Gavilán, C.G. An Overview on Fecal Branched Short-Chain Fatty Acids Along Human Life and as Related with Body Mass Index: Associated Dietary and Anthropometric Factors. Front. Microbiol. 2020, 11, 973.
➤ Get "Smart Citations"
(hover to see more details)
.
Interestingly, BCFA levels in fecal samples exhibit an inverse connection with fiber intake, particularly insoluble fiber.
Elevated BCFA levels have been associated with depression and other psychiatric conditions, potentially mediated through vagal afferent nerve signaling.
Additionally, BCFA, especially isobutyrate, have been linked to unfavorable serum lipid profiles in subjects with hypercholesterolemia ✅ Trusted Source
➤ Go to source
Granado-Serrano, A.B.; Martín-Garí, M.; Sánchez, V.; Riart Solans, M.; Berdún, R.; Ludwig, I.A.; Rubió, L.; Vilaprinyó, E.; Portero-Otín, M.; Serrano, J.C.E. Faecal bacterial and short-chain fatty acids signature in hypercholesterolemia. Sci. Rep. 2019, 9, 1772.
➤ Get "Smart Citations"
(hover to see more details)
.
Such higher BCFA levels may correspond to increased protein consumption and reduced dietary fiber intake, factors known to contribute to negative health outcomes and age-related complications ✅ Trusted Source
➤ Go to source
Rios-Covian, D.; González, S.; Nogacka, A.M.; Arboleya, S.; Salazar, N.; Gueimonde, M.; de Los Reyes-Gavilán, C.G. An Overview on Fecal Branched Short-Chain Fatty Acids Along Human Life and as Related with Body Mass Index: Associated Dietary and Anthropometric Factors. Front. Microbiol. 2020, 11, 973.
➤ Get "Smart Citations"
(hover to see more details)
.
DF Fermentation Products: Post-biotics
Recent nomenclature labels the byproducts of DF fermentation as "post-biotics."
In adults, the primary products of DF fermentation include SCFA and certain gases like CO2, CH4, and H2, which can be absorbed by the host or excreted ✅ Trusted Source
➤ Go to source
Topping, D.L.; Clifton, P.M. Short-Chain Fatty Acids and Human Colonic Function: Roles of Resistant Starch and Nonstarch Polysaccharides. Physiol. Rev. 2001, 81, 1031–1064.
➤ Get "Smart Citations"
(hover to see more details)
.
SCFA production in the colon coincides with the consumption of ammonia, hydrogen sulfide (H2S), and BCFA by gut bacteria in the synthesis of microbial cell components.
Consequently, the reduction of these metabolites may partly account for the health benefits attributed to SCFA ✅ Trusted Source
➤ Go to source
Sakata, T. Pitfalls in short-chain fatty acid research: A methodological review. Anim. Sci. J. 2019, 90, 3–13.
➤ Get "Smart Citations"
(hover to see more details)
.
Notably, ammonia has been associated with negative health outcomes, including neurotoxicity and hepatotoxicity, as well as increased intestinal permeability, loss of tight junction proteins, and elevated pro-inflammatory cytokines, as observed in animal studies ✅ Trusted Source
➤ Go to source
Dasarathy, S.; Mookerjee, R.P.; Rackayova, V.; Rangroo Thrane, V.; Vairappan, B.; Ott, P.; Rose, C.F. Ammonia toxicity: From head to toe? Metab. Brain Dis. 2017, 32, 529–538.
➤ Get "Smart Citations"
(hover to see more details)
.
H2S, hydrogen disulfide, when abnormally produced, has been linked to neurological, cardiovascular, and metabolic diseases ✅ Trusted Source
➤ Go to source
Di Masi, A.; Ascenzi, P. H2S: A “double face” molecule in health and disease. BioFactors 2013, 39, 186–196.
➤ Get "Smart Citations"
(hover to see more details)
.
How Individual Differences in Gut Microbiota
Dietary fiber (DF) impacts people differently, but understanding this variation is challenging.
Various factors, including genetics and food matrix properties, contribute to the diverse responses observed in nutritional studies ✅ Trusted Source
➤ Go to source
Carlson, J.; Esparza, J.; Swan, J.; Taussig, D.; Combs, J.; Slavin, J. In vitro analysis of partially hydrolyzed guar gum fermentation differences between six individuals. Food Funct. 2016, 7, 1833–1838.
➤ Get "Smart Citations"
(hover to see more details)
✅ Trusted Source
➤ Go to source
Potter, T.; Vieira, R.; de Roos, B. Perspective: Application of N-of-1 Methods in Personalized Nutrition Research. Adv. Nutr. 2021, 12, 579–589.
➤ Get "Smart Citations"
(hover to see more details)
.
Bioavailability of DF-derived metabolites, like vitamins, is influenced by the SLAMENGHI factors (molecular species, linkage, amount, matrix, absorption effectors, nutrition status, genetics, and host-related factors) ✅ Trusted Source
➤ Go to source
Jakobsen, J.; Melse-Boonstra, A.; Rychlik, M. Challenges to Quantify Total Vitamin Activity: How to Combine the Contribution of Diverse Vitamers? Curr. Dev. Nutr. 2019, 3, nzz086.
➤ Get "Smart Citations"
(hover to see more details)
.
Inconsistencies also arise from differing DF definitions across studies.
To address this complexity, a systems analysis approach is recommended, similar to clinical oncology ✅ Trusted Source
➤ Go to source
Yurkovich, J.T.; Tian, Q.; Price, N.D.; Hood, L. A systems approach to clinical oncology uses deep phenotyping to deliver personalized care. Nature 2020, 17, 183–194.
➤ Get "Smart Citations"
(hover to see more details)
.
It's crucial to recognize the baseline variation in the effects of DF consumption on health outcomes, related to individual gut microbiota (enterotype) ✅ Trusted Source
➤ Go to source
Arumugam, M.; Raes, J.; Pelletier, E.; le Paslier, D.; Yamada, T.; Mende, D.R.; Fernandes, G.R.; Tap, J.; Bruls, T.; Batto, J.-M.; et al. Enterotypes of the human gut microbiome. Nature 2011, 473, 174–180.
➤ Get "Smart Citations"
(hover to see more details)
✅ Trusted Source
➤ Go to source
Christensen, L.; Roager, H.M.; Astrup, A.; Hjorth, M.F. Microbial enterotypes in personalized nutrition and obesity management. Am. J. Clin. Nutr. 2018, 108, 645–651.
➤ Get "Smart Citations"
(hover to see more details)
.
The impact of DF on metabolism and health outcomes varies based on one's unique gut microbiota composition ✅ Trusted Source
➤ Go to source
Zeevi, D.; Korem, T.; Zmora, N.; Israeli, D.; Rothschild, D.; Weinberger, A.; Ben-Yacov, O.; Lador, D.; Avnit-Sagi, T.; Lotan-Pompan, M.; et al. Personalized Nutrition by Prediction of Glycemic Responses. Cell 2015, 163, 1079–1094.
➤ Get "Smart Citations"
(hover to see more details)
.
While DF is generally beneficial, it may lead to discomfort in those with low prior DF intake.
The following sections delve into three layers of inter-individual variability (enterotypes, genotypes, and phenotypes) among healthy adults ✅ Trusted Source
➤ Go to source
Christodoulides, S.; Dimidi, E.; Fragkos, K.C.; Farmer, A.D.; Whelan, K.; Scott, S.M. Systematic review with meta-analysis: Effect of fibre supplementation on chronic idiopathic constipation in adults. Aliment. Pharmacol. Ther. 2016, 44, 103–116.
➤ Get "Smart Citations"
(hover to see more details)
✅ Trusted Source
➤ Go to source
Li, J.; Jia, H.; Cai, X.; Zhong, H.; Feng, Q.; Sunagawa, S.; Arumugam, M.; Kultima, J.R.; Prifti, E.; Nielsen, T.; et al. An integrated catalog of reference genes in the human gut microbiome. Nat. Biotechnol. 2014, 32, 834–841.
➤ Get "Smart Citations"
(hover to see more details)
.
Gut Microbiota and Factors Influencing It
Gut microbiota composition is shaped by numerous factors.
Host-related aspects like age, genetics, immune status, and medication use, along with environmental elements such as geography, diet, and pollutants, influence the gut microbiota ✅ Trusted Source
➤ Go to source
Spor, A.; Koren, O.; Ley, R. Unravelling the effects of the environment and host genotype on the gut microbiome. Nat. Rev. Microbiol. 2011, 9, 279–290.
➤ Get "Smart Citations"
(hover to see more details)
✅ Trusted Source
➤ Go to source
Benson, A.K.; Kelly, S.A.; Legge, R.; Ma, F.; Low, S.J.; Kim, J.; Zhang, M.; Oh, P.L.; Nehrenberg, D.; Hua, K.; et al. Individuality in gut microbiota composition is a complex polygenic trait shaped by multiple environmental and host genetic factors. Proc. Natl. Acad. Sci. USA 2010, 107, 18933–18938.
➤ Get "Smart Citations"
(hover to see more details)
✅ Trusted Source
➤ Go to source
Rothschild, D.; Weissbrod, O.; Barkan, E.; Kurilshikov, A.; Korem, T.; Zeevi, D.; Costea, P.I.; Godneva, A.; Kalka, I.N.; Bar, N.; et al. Environment dominates over host genetics in shaping human gut microbiota. Nature 2018, 555, 210–215.
➤ Get "Smart Citations"
(hover to see more details)
.
Microbial factors, including substrate competition, metabolic collaboration, and species interactions, also play a pivotal role, as do the microbial environment's local pH, redox potential, and quorum sensing.
The gut microbiota mainly comprises Firmicutes, Bacteroidetes, Proteobacteria, Verrucobacteria, Actinobacteria, and Fusobacteria, with Bacteroidetes and Firmicutes making up 70% of the total composition in adulthood ✅ Trusted Source
➤ Go to source
Iebba, V.; Totino, V.; Gagliardi, A.; Santangelo, F.; Cacciotti, F.; Trancassini, M.; Mancini, C.; Cicerone, C.; Corazziari, E.; Pantanella, F.; et al. Eubiosis and dysbiosis: The two sides of the microbiota. New Microbiol. 2016, 39, 1–12. .
Early in life, Proteobacteria and Actinobacteria dominate, influenced by delivery and feeding methods in infancy.
Approximately 6.6% of microbial taxa are heritable, while around 48.6% are influenced by cohabitation ✅ Trusted Source
➤ Go to source
Goodrich, J.K.; Davenport, E.R.; Waters, J.L.; Clark, A.G.; Ley, R.E. Cross-species comparisons of host genetic associations with the microbiome. Science 2016, 352, 532–535.
➤ Get "Smart Citations"
(hover to see more details)
.
Specific bacteria are involved in the production of short-chain fatty acids (SCFA) through dietary fiber fermentation.
SCFA impacts gut microbiota and health outcomes ✅ Trusted Source
➤ Go to source
Macfarlane, G.T.; Macfarlane, S. Bacteria, Colonic Fermentation, and Gastrointestinal Health. J. AOAC Int. 2012, 95, 50–60.
➤ Get "Smart Citations"
(hover to see more details)
.
Glycans and GOS have been shown to increase Bifidobacterium and Lactobacillus abundance, as well as faecal butyrate concentration ✅ Trusted Source
➤ Go to source
So, D.; Whelan, K.; Rossi, M.; Morrison, M.; Holtmann, G.; Kelly, J.T.; Shanahan, E.R.; Staudacher, H.M.; Campbell, K.L. Dietary fiber intervention on gut microbiota composition in healthy adults: A systematic review and meta-analysis. Am. J. Clin. Nutr. 2018, 107, 965–983.
➤ Get "Smart Citations"
(hover to see more details)
.
Bacteroidetes mainly produce acetate and propionate, while Firmicutes tend to generate butyrate, which may influence the host's inflammatory state.
Enterotypes, which represent compositional gradients rather than discrete clusters, have been proposed, highlighting distinct genus-level compositions ✅ Trusted Source
➤ Go to source
Costea, P.I.; Hildebrand, F.; Arumugam, M.; Bäckhed, F.; Blaser, M.J.; Bushman, F.D.; Willem, M.S.; Fraser, C.M.; Hattori, M.; Huttenhower, C.; et al. Enterotypes in the landscape of gut microbial community composition. Nat. Microbiol. 2018, 3, 8–16.
➤ Get "Smart Citations"
(hover to see more details)
.
Prevotella, a key player in one enterotype, is associated with various health outcomes, including inflammation and colitis ✅ Trusted Source
➤ Go to source
Amat, S.; Lantz, H.; Munyaka, P.M.; Willing, B.P. Prevotella in Pigs: The Positive and Negative Associations with Production and Health. Microorganisms 2020, 8, 1584.
➤ Get "Smart Citations"
(hover to see more details)
.
However, its role in diseases like autism, rheumatoid arthritis, and HIV remains inconclusive ✅ Trusted Source
➤ Go to source
Duvallet, C.; Gibbons, S.M.; Gurry, T.; Irizarry, R.A.; Alm, E.J. Meta-analysis of gut microbiome studies identifies disease-specific and shared responses. Nat. Commun. 2017, 8, 1784.
➤ Get "Smart Citations"
(hover to see more details)
.
Akkermansia muciniphila, a mucin-degrading bacterium, contributes to mucus layer regulation ✅ Trusted Source
➤ Go to source
Derrien, M.; Belzer, C.; de Vos, W.M. Akkermansia muciniphila and its role in regulating host functions. Microb. Pathog. 2017, 106, 171–181.
➤ Get "Smart Citations"
(hover to see more details)
.
Akkermansia's abundance decreases with age and has been associated with various health conditions, such as type 1 diabetes ✅ Trusted Source
➤ Go to source
Bodogai, M.; O’Connell, J.; Kim, K.; Kim, Y.; Moritoh, K.; Chen, C.; Gusev, F.; Vaughan, K.; Shulzhenko, N.; Mattison, J.A.; et al. Commensal bacteria contribute to insulin resistance in aging by activating innate B1a cells. Sci. Transl. Med. 2018, 10, eaat4271.
➤ Get "Smart Citations"
(hover to see more details)
.
Dysbiosis, an imbalanced gut microbiota, can develop from an early age and may lead to inflammatory or hypotrophic dysbiosis ✅ Trusted Source
➤ Go to source
Parkin, K.; Christophersen, C.T.; Verhasselt, V.; Cooper, M.N.; Martino, D. Risk Factors for Gut Dysbiosis in Early Life. Microorganisms 2021, 9, 2066.
➤ Get "Smart Citations"
(hover to see more details)
.
Hypotrophic dysbiosis can predispose individuals to inflammatory states and diseases like Giardia lamblia infection-induced diarrhea ✅ Trusted Source
➤ Go to source
Barash, N.R.; Maloney, J.G.; Singer, S.M.; Dawson, S.C. Giardia Alters Commensal Microbial Diversity throughout the Murine Gut. Infect. Immun. 2017, 85, e00948-16.
➤ Get "Smart Citations"
(hover to see more details)
.
Genes and Gut Microbiota
The relationship between the human genome and the microbiome is intricate and ever-changing ✅ Trusted Source
➤ Go to source
Bordenstein, S.R.; Theis, K.R. Host Biology in Light of the Microbiome: Ten Principles of Holobionts and Hologenomes. PLoS Biol. 2015, 13, e1002226.
➤ Get "Smart Citations"
(hover to see more details)
.
While the composition of the gut microbiome can shift during a lifetime, influenced by factors like diet, lifestyle choices, and location ✅ Trusted Source
➤ Go to source
Sommer, F.; Bäckhed, F. The gut microbiota—Masters of host development and physiology. Nat. Rev. Microbiol. 2013, 11, 227–238.
➤ Get "Smart Citations"
(hover to see more details)
✅ Trusted Source
➤ Go to source
Li, J.; Jia, H.; Cai, X.; Zhong, H.; Feng, Q.; Sunagawa, S.; Arumugam, M.; Kultima, J.R.; Prifti, E.; Nielsen, T.; et al. An integrated catalog of reference genes in the human gut microbiome. Nat. Biotechnol. 2014, 32, 834–841.
➤ Get "Smart Citations"
(hover to see more details)
, the human genome remains relatively stable, with potential epigenetic changes due to environmental exposure ✅ Trusted Source
➤ Go to source
Goodrich, J.K.; Davenport, E.R.; Clark, A.G.; Ley, R.E. The Relationship Between the Human Genome and Microbiome Comes into View. Annu. Rev. Genet. 2017, 51, 413–433.
➤ Get "Smart Citations"
(hover to see more details)
✅ Trusted Source
➤ Go to source
Telenti, A.; Jiang, X. Treating medical data as a durable asset. Nat. Genet. 2020, 52, 1005–1010.
➤ Get "Smart Citations"
(hover to see more details)
✅ Trusted Source
➤ Go to source
Wainschtein, P.; Jain, D.; Zheng, Z.; Cupples, L.A.; Shadyab, A.H.; McKnight, B.; Shoemaker, B.M.; Mitchell, B.D.; Psaty, B.M.; Kooperberg, C.; et al. Recovery of trait heritability from whole genome sequence data. bioRxiv 2019.
➤ Get "Smart Citations"
(hover to see more details)
.
In the realm of nutrigenetics, the focus is on how an individual's genetic makeup, including copy number variations (CNVs) and single nucleotide polymorphisms (SNPs), can impact the fate of different foods in terms of absorption, distribution, metabolism, or excretion (ADME) patterns (Table 3).
Recent discoveries have identified CNVs and SNPs in various regions of the genome as drivers of differences in individuals' physical traits and health outcomes.
Polygenic risk scores, which combine multiple genetic factors, have been linked to a wide range of human conditions ✅ Trusted Source
➤ Go to source
Billingsley, K.J.; Barbosa, I.A.; Bandrés-Ciga, S.; Quinn, J.P.; Bubb, V.J.; Deshpande, C.; Botia, J.A.; Reynolds, R.H.; Zhang, D.; Simpson, M.A.; et al. Mitochondria function associated genes contribute to Parkinson’s Disease risk and later age at onset. NPJ Parkinson’s Dis. 2019, 5, 8.
➤ Get "Smart Citations"
(hover to see more details)
✅ Trusted Source
➤ Go to source
Natarajan, P.; Young, R.; Stitziel, N.O.; Padmanabhan, S.; Baber, U.; Mehran, R.; Sartori, S.; Fuster, V.; Reilly, D.F.; Butterworth, A.; et al. Polygenic Risk Score Identifies Subgroup with Higher Burden of Atherosclerosis and Greater Relative Benefit from Statin Therapy in the Primary Prevention Setting. Circulation 2017, 135, 2091–2101.
➤ Get "Smart Citations"
(hover to see more details)
.
When we consider homeostasis at the holobiont level, where the host genome and microbiome interact ✅ Trusted Source
➤ Go to source
Bordenstein, S.R.; Theis, K.R. Host Biology in Light of the Microbiome: Ten Principles of Holobionts and Hologenomes. PLoS Biol. 2015, 13, e1002226.
➤ Get "Smart Citations"
(hover to see more details)
✅ Trusted Source
➤ Go to source
Levy, M.; Thaiss, C.A.; Elinav, E. Metabolites: Messengers between the microbiota and the immune system. Genes Dev. 2016, 30, 1589–1597.
➤ Get "Smart Citations"
(hover to see more details)
, polygenic risk becomes particularly relevant ✅ Trusted Source
➤ Go to source
Choi, S.W.; Mak, T.S.-H.; O’Reilly, P.F. Tutorial: A guide to performing polygenic risk score analyses. Nat. Protoc. 2020, 15, 2759–2772.
➤ Get "Smart Citations"
(hover to see more details)
.
Twin studies have suggested that specific SNPs can influence microbiota colonization from the earliest days of life, acting as a foundation for achieving optimal homeostasis in an ever-changing environment ✅ Trusted Source
➤ Go to source
Esworthy, R.S.; Smith, D.D.; Chu, F.-F. A Strong Impact of Genetic Background on Gut Microflora in Mice. Int. J. Inflamm. 2010, 2010, 986046.
➤ Get "Smart Citations"
(hover to see more details)
✅ Trusted Source
➤ Go to source
Blekhman, R.; Goodrich, J.K.; Huang, K.; Sun, Q.; Bukowski, R.; Bell, J.T.; Spector, T.D.; Keinan, A.; Ley, R.E.; Gevers, D.; et al. Host genetic variation impacts microbiome composition across human body sites. Genome Biol. 2015, 16, 191.
➤ Get "Smart Citations"
(hover to see more details)
.
Certain SNPs, such as those related to G-protein coupled cellular receptors (GPCR41, GPCR43, GPCR109A), transporter genes like MCT and SMCT, effector genes like MUC2 (involved in mucus layer production), and regulatory genes like NRF2 (which regulates antioxidant defense proteins), may individually or collectively predispose individuals to various health outcomes.
Exploring elderly populations, researchers have identified genes associated with inflammatory responses (IL-6, IL-10, and the IL-1 cluster), genes involved in the insulin/IGF1 pathway, and genes related to oxidative stress management (PON1) as correlated with extreme old age ✅ Trusted Source
➤ Go to source
Franceschi, C.; Olivieri, F.; Marchegiani, F.; Cardelli, M.; Cavallone, L.; Capri, M.; Salvioli, S.; Valensin, S.; Benedictis, G.D.; Iorio, A.D.; et al. Genes involved in immune response/inflammation, IGF1/insulin pathway and response to oxidative stress play a major role in the genetics of human longevity: The lesson of centenarians. Mech. Ageing Dev. 2005, 126, 351–361.
➤ Get "Smart Citations"
(hover to see more details)
.
While bacterial colonization in early life is crucial for various aspects of immune development, the host's genetic background can modulate the impact of bacteria.
For example, certain genetic modifications, like programmed cell death (PD1) knockout in mice, can lead to changes in microbiota profiles, affecting the abundance of specific bacterial genera ✅ Trusted Source
➤ Go to source
Kawamoto, S.; Tran, T.H.; Maruya, M.; Suzuki, K.; Doi, Y.; Tsutsui, Y.; Kato, L.M.; Fagarasan, S. The Inhibitory Receptor PD-1 Regulates IgA Selection and Bacterial Composition in the Gut. Science 2012, 336, 485–489.
➤ Get "Smart Citations"
(hover to see more details)
.
The secretion of immunoglobulin A (IgA) is crucial for the colonization of beneficial bacteria and defense against pathogens.
This highlights the intricate interplay between the host genome and the microbiome, where commensal bacteria require IgA coating for colonization, while the same coating triggers immune responses against pathogenic bacteria ✅ Trusted Source
➤ Go to source
Donaldson, G.P.; Ladinsky, M.S.; Yu, K.B.; Sanders, J.G.; Yoo, B.B.; Chou, W.C.; Conner, M.E.; Earl, A.M.; Knight, R.; Bjorkman, P.J.; et al. Gut microbiota utilize immunoglobulin A for mucosal colonization. Science 2018, 360, 795–800.
➤ Get "Smart Citations"
(hover to see more details)
✅ Trusted Source
➤ Go to source
Nakajima, A.; Vogelzang, A.; Maruya, M.; Miyajima, M.; Murata, M.; Son, A.; Kuwahara, T.; Tsuruyama, T.; Yamada, S.; Matsuura, M.; et al. IgA regulates the composition and metabolic function of gut microbiota by promoting symbiosis between bacteria. J. Exp. Med. 2018, 215, 2019–2034.
➤ Get "Smart Citations"
(hover to see more details)
.
Microbial acetate in the gut even regulates IgA reactivity to commensal bacteria, influencing microbiota species and colonization in the colon ✅ Trusted Source
➤ Go to source
Takeuchi, T.; Miyauchi, E.; Kanaya, T.; Kato, T.; Nakanishi, Y.; Watanabe, T.; Kitami, T.; Taida, T.; Sasaki, T.; Negishi, H.; et al. Acetate differentially regulates IgA reactivity to commensal bacteria. Nature 2021, 595, 560–564.
➤ Get "Smart Citations"
(hover to see more details)
.
Antimicrobial peptides (AMPs) produced by intraepithelial cells (IEC) in the gut are another piece of the puzzle.
These peptides, like α-defensins and β-defensin 1, are rapidly activated by IL-22 and IL-17 to inactivate invading microorganisms.
AMPs not only help maintain the separation between the host and microorganisms but also affect microbial composition ✅ Trusted Source
➤ Go to source
Liang, S.C.; Tan, X.-Y.; Luxenberg, D.P.; Karim, R.; Dunussi-Joannopoulos, K.; Collins, M.; Fouser, L.A. Interleukin (IL)-22 and IL-17 are coexpressed by Th17 cells and cooperatively enhance expression of antimicrobial peptides. J. Exp. Med. 2006, 203, 2271–2279.
➤ Get "Smart Citations"
(hover to see more details)
.
Studies with mice deficient in genes like MYD88, NOD2, or those transgenic for α-defensin 5 have shown altered microbiota compositions ✅ Trusted Source
➤ Go to source
Kawai, T.; Adachi, O.; Ogawa, T.; Takeda, K.; Akira, S. Unresponsiveness of MyD88-deficient mice to endotoxin. Immunity 1999, 11, 115–122.
➤ Get "Smart Citations"
(hover to see more details)
✅ Trusted Source
➤ Go to source
Petnicki-Ocwieja, T.; Hrncir, T.; Liu, Y.-J.; Biswas, A.; Hudcovic, T.; Tlaskalova-Hogenova, H.; Kobayashi, K.S. Nod2 is required for the regulation of commensal microbiota in the intestine. Proc. Natl. Acad. Sci. USA 2009, 106, 15813–15818.
➤ Get "Smart Citations"
(hover to see more details)
✅ Trusted Source
➤ Go to source
Knights, D.; Silverberg, M.S.; Weersma, R.K.; Gevers, D.; Dijkstra, G.; Huang, H.; Tyler, A.D.; van Sommeren, S.; Imhann, F.; Stempak, J.M.; et al. Complex host genetics influence the microbiome in inflammatory bowel disease. Genome Med. 2014, 6, 107.
➤ Get "Smart Citations"
(hover to see more details)
✅ Trusted Source
➤ Go to source
Salzman, N.H.; Hung, K.; Haribhai, D.; Chu, H.; Karlsson-Sjöberg, J.; Amir, E.; Teggatz, P.; Barman, M.; Hayward, M.; Eastwood, D.; et al. Enteric defensins are essential regulators of intestinal microbial ecology. Nat. Immunol. 2010, 11, 76–82.
➤ Get "Smart Citations"
(hover to see more details)
.
When examining Alzheimer's disease, the APOε4 allele has been linked to higher levels of pro-inflammatory bacteria, while the protective APOε2 allele correlates with SCFA-producing bacteria ✅ Trusted Source
➤ Go to source
Salzman, N.H.; Hung, K.; Haribhai, D.; Chu, H.; Karlsson-Sjöberg, J.; Amir, E.; Teggatz, P.; Barman, M.; Hayward, M.; Eastwood, D.; et al. Enteric defensins are essential regulators of intestinal microbial ecology. Nat. Immunol. 2010, 11, 76–82.
➤ Get "Smart Citations"
(hover to see more details)
.
SCFAs have even been found to inhibit the aggregation of amyloid β (Aβ), a hallmark of Alzheimer's disease, in vitro.
Mutations in MEFV, associated with familial Mediterranean fever, have also demonstrated the host's ability to modulate microbial composition.
This suggests that the host genome and microbiome structure are functionally linked, impacting responses to dietary fiber both directly (via SCFA metabolism) and indirectly (via microbiome modulation) ✅ Trusted Source
➤ Go to source
Goodrich, J.K.; Davenport, E.R.; Beaumont, M.; Jackson, M.A.; Knight, R.; Ober, C.; Spector, T.D.; Bell, J.T.; Clark, A.G.; Ley, R.E. Genetic Determinants of the Gut Microbiome in UK Twins. Cell Host Microbe 2016, 19, 731–743.
➤ Get "Smart Citations"
(hover to see more details)
.
Genetic Symphony of Short Chain Fatty Acids and Gut Microbiota
In the intricate world of nutrigenomics, where nutrients orchestrate gene expression, Short Chain Fatty Acids (SCFAs) take center stage.
SCFAs can directly influence genetic expression by modulating histone deacetylases ✅ Trusted Source
➤ Go to source
Chang, P.V.; Hao, L.; Offermanns, S.; Medzhitov, R. The microbial metabolite butyrate regulates intestinal macrophage function via histone deacetylase inhibition. Proc. Natl. Acad. Sci. USA 2014, 111, 2247–2252.
➤ Get "Smart Citations"
(hover to see more details)
✅ Trusted Source
➤ Go to source
Zimmerman, M.A.; Singh, N.; Martin, P.M.; Thangaraju, M.; Ganapathy, V.; Waller, J.L.; Shi, H.; Robertson, K.D.; Munn, D.H.; Liu, K. Butyrate suppresses colonic inflammation through HDAC1-dependent Fas upregulation and Fas-mediated apoptosis of T cells. Am. J. Physiol.-Gastrointest. Liver Physiol. 2012, 302, G1405–G1415.
➤ Get "Smart Citations"
(hover to see more details)
✅ Trusted Source
➤ Go to source
Park, J.; Kim, M.; Kang, S.G.; Jannasch, A.H.; Cooper, B.; Patterson, J.; Kim, C.H. Short-chain fatty acids induce both effector and regulatory T cells by suppression of histone deacetylases and regulation of the mTOR–S6K pathway. Mucosal Immunol. 2015, 8, 80–93.
➤ Get "Smart Citations"
(hover to see more details)
✅ Trusted Source
➤ Go to source
Tan, J.; McKenzie, C.; Potamitis, M.; Thorburn, A.N.; Mackay, C.R.; Macia, L. The Role of Short-Chain Fatty Acids in Health and Disease. In Advances in Immunology; Elsevier: Amsterdam, The Netherlands, 2014; Volume 121, pp. 91–112. .
Over time, these epigenetic changes can shape an individual's observable characteristics, known as their phenotype.
Phenotypes are often measured through various parameters, like age, body mass index (BMI), or blood counts, and their interpretation can vary depending on the type of study, be it epidemiological, clinical, or mechanistic ✅ Trusted Source
➤ Go to source
Broek, T.J.V.D.; Bakker, G.C.M.; Rubingh, C.M.; Bijlsma, S.; Stroeve, J.H.M.; Ommen, B.V.; Erk, M.J.V.; Wopereis, S. Ranges of phenotypic flexibility in healthy subjects. Genes Nutr. 2017, 12, 32.
➤ Get "Smart Citations"
(hover to see more details)
✅ Trusted Source
➤ Go to source
Stroeve, J.H.M.; Wietmarschen, H.V.; Kremer, B.H.A.; Ommen, B.V.; Wopereis, S. Phenotypic flexibility as a measure of health: The optimal nutritional stress response test. Genes Nutr. 2015, 10, 12–33.
➤ Get "Smart Citations"
(hover to see more details)
✅ Trusted Source
➤ Go to source
Van Ommen, B.; van der Greef, J.; Ordovas, J.M.; Daniel, H. Phenotypic flexibility as key factor in the human nutrition and health relationship. Genes Nutr. 2014, 9, 423.
➤ Get "Smart Citations"
(hover to see more details)
.
This variability is influenced by genetic predisposition and the individual's ability to maintain balance in the face of environmental stressors ✅ Trusted Source
➤ Go to source
Broek, T.J.V.D.; Bakker, G.C.M.; Rubingh, C.M.; Bijlsma, S.; Stroeve, J.H.M.; Ommen, B.V.; Erk, M.J.V.; Wopereis, S. Ranges of phenotypic flexibility in healthy subjects. Genes Nutr. 2017, 12, 32.
➤ Get "Smart Citations"
(hover to see more details)
✅ Trusted Source
➤ Go to source
Stroeve, J.H.M.; Wietmarschen, H.V.; Kremer, B.H.A.; Ommen, B.V.; Wopereis, S. Phenotypic flexibility as a measure of health: The optimal nutritional stress response test. Genes Nutr. 2015, 10, 12–33.
➤ Get "Smart Citations"
(hover to see more details)
✅ Trusted Source
➤ Go to source
Van Ommen, B.; van der Greef, J.; Ordovas, J.M.; Daniel, H. Phenotypic flexibility as key factor in the human nutrition and health relationship. Genes Nutr. 2014, 9, 423.
➤ Get "Smart Citations"
(hover to see more details)
.
It's essential to understand that these associations at the phenotype level, such as the link between BMI and metabolic issues or glycated hemoglobin levels and diabetes progression, are influenced by a complex interplay of genetics, microbiome, and diet.
Some genotypes may lead to increased inflammatory function, which is beneficial in defending against infections.
However, when a low-grade chronic inflammation becomes undesirable, as in Non-Communicable Diseases (NCDs) and aging, these genotypes can have detrimental effects ✅ Trusted Source
➤ Go to source
Kotas, M.E.; Medzhitov, R. Homeostasis, Inflammation, and Disease Susceptibility. Cell 2015, 160, 816–827.
➤ Get "Smart Citations"
(hover to see more details)
✅ Trusted Source
➤ Go to source
Franceschi, C.; Olivieri, F.; Marchegiani, F.; Cardelli, M.; Cavallone, L.; Capri, M.; Salvioli, S.; Valensin, S.; Benedictis, G.D.; Iorio, A.D.; et al. Genes involved in immune response/inflammation, IGF1/insulin pathway and response to oxidative stress play a major role in the genetics of human longevity: The lesson of centenarians. Mech. Ageing Dev. 2005, 126, 351–361.
➤ Get "Smart Citations"
(hover to see more details)
✅ Trusted Source
➤ Go to source
Duncan, B.B.; Schmidt, M.I.S.; Pankow, J.S.; Ballantyne, C.M.; Couper, D.; Vigo, A.; Hoogeveen, R.; Folsom, A.R.; Heiss, G. Low-Grade Systemic Inflammation and the Development of Type 2 Diabetes. Diabetes 2003, 52, 1799–1805.
➤ Get "Smart Citations"
(hover to see more details)
.
Even genes unrelated to immune function can play a crucial role in achieving holobiont homeostasis, with implications for NCDs and aging progression, as seen with genes like FUT-2 and AMY1 ✅ Trusted Source
➤ Go to source
Poole, A.C.; Goodrich, J.K.; Youngblut, N.D.; Luque, G.G.; Ruaud, A.; Sutter, J.L.; Waters, J.L.; Shi, Q.; El-Hadidi, M.; Johnson, L.M.; et al. Human Salivary Amylase Gene Copy Number Impacts Oral and Gut Microbiomes. Cell Host Microbe 2019, 25, 553–564.e557.
➤ Get "Smart Citations"
(hover to see more details)
✅ Trusted Source
➤ Go to source
Tong, M.; McHardy, I.; Ruegger, P.; Goudarzi, M.; Kashyap, P.C.; Haritunians, T.; Li, X.; Graeber, T.G.; Schwager, E.; Huttenhower, C.; et al. Reprograming of gut microbiome energy metabolism by the FUT2 Crohn’s disease risk polymorphism. ISME J. 2014, 8, 2193–2206.
➤ Get "Smart Citations"
(hover to see more details)
.
Centenarians, individuals who live beyond 100 years without significant chronic diseases, offer intriguing insights.
Studies suggest that centenarians have distinctive gut microbiome signatures compared to both young adults (20-40 years old) and the general elderly population (60-80 years old) ✅ Trusted Source
➤ Go to source
Biagi, E.; Candela, M.; Turroni, S.; Garagnani, P.; Franceschi, C.; Brigidi, P. Ageing and gut microbes: Perspectives for health maintenance and longevity. Pharmacol. Res. 2013, 1, 11–20.
➤ Get "Smart Citations"
(hover to see more details)
✅ Trusted Source
➤ Go to source
Biagi, E.; Nylund, L.; Candela, M.; Ostan, R.; Bucci, L.; Pini, E.; Nikkïla, J.; Monti, D.; Satokari, R.; Franceschi, C.; et al. Through Ageing, and Beyond: Gut Microbiota and Inflammatory Status in Seniors and Centenarians. PLoS ONE 2010, 5, e10667.
➤ Get "Smart Citations"
(hover to see more details)
✅ Trusted Source
➤ Go to source
Biagi, E.; Candela, M.; Fairweather-Tait, S.; Franceschi, C.; Brigidi, P. Ageing of the human metaorganism: The microbial counterpart. Age 2012, 34, 247–267.
➤ Get "Smart Citations"
(hover to see more details)
.
These variations could be attributed to specific gene polymorphisms.
For instance, the FUT2 gene, responsible for regulating glycans in mucosal tissues, has links to conditions like Crohn's disease ✅ Trusted Source
➤ Go to source
Tong, M.; McHardy, I.; Ruegger, P.; Goudarzi, M.; Kashyap, P.C.; Haritunians, T.; Li, X.; Graeber, T.G.; Schwager, E.; Huttenhower, C.; et al. Reprograming of gut microbiome energy metabolism by the FUT2 Crohn’s disease risk polymorphism. ISME J. 2014, 8, 2193–2206.
➤ Get "Smart Citations"
(hover to see more details)
.
Enterotyping has shown that the secretor phenotype, associated with Firmicutes, Akkermansia, and Ruminococcus spp., may be influenced by certain genes ✅ Trusted Source
➤ Go to source
Wacklin, P.; Tuimala, J.; Nikkilä, J.; Sebastian, T.; Mäkivuokko, H.; Alakulppi, N.; Laine, P.; Rajilic-Stojanovic, M.; Paulin, L.; de Vos, W.M.; et al. Faecal Microbiota Composition in Adults Is Associated with the FUT2 Gene Determining the Secretor Status. PLoS ONE 2014, 9, e94863.
➤ Get "Smart Citations"
(hover to see more details)
.
This underscores the idea that genes not directly linked to immune function can significantly impact health outcomes, including NCDs and survival into old age ✅ Trusted Source
➤ Go to source
Barzilai, N.; Huffman, D.M.; Muzumdar, R.H.; Bartke, A. The Critical Role of Metabolic Pathways in Aging. Diabetes 2012, 61, 1315–1322.
➤ Get "Smart Citations"
(hover to see more details)
.
In mice, genotype plays a recognized role in microbial colonization ✅ Trusted Source
➤ Go to source
Esworthy, R.S.; Smith, D.D.; Chu, F.-F. A Strong Impact of Genetic Background on Gut Microflora in Mice. Int. J. Inflamm. 2010, 2010, 986046.
➤ Get "Smart Citations"
(hover to see more details)
.
The timing of meals, known as chrononutrition, can also lead to significant changes in gut microbiome composition, immune responses, and metabolic conditions, including Type 2 Diabetes (T2D), Cardiovascular Disease (CVD), and psychological well-being ✅ Trusted Source
➤ Go to source
Henry, C.J.; Kaur, B.; Quek, R.Y.C. Chrononutrition in the management of diabetes. Nutr. Diabetes 2020, 10, 6.
➤ Get "Smart Citations"
(hover to see more details)
✅ Trusted Source
➤ Go to source
Papakonstantinou, E.; Oikonomou, C.; Nychas, G.; Dimitriadis, G.D. Effects of Diet, Lifestyle, Chrononutrition and Alternative Dietary Interventions on Postprandial Glycemia and Insulin Resistance. Nutrients 2022, 14, 823.
➤ Get "Smart Citations"
(hover to see more details)
✅ Trusted Source
➤ Go to source
Katsi, V.; Papakonstantinou, I.P.; Soulaidopoulos, S.; Katsiki, N.; Tsioufis, K. Chrononutrition in Cardiometabolic Health. J. Clin. Med. 2022, 11, 296.
➤ Get "Smart Citations"
(hover to see more details)
✅ Trusted Source
➤ Go to source
Mohd Azmi, N.A.S.; Juliana, N.; Mohd Fahmi Teng, N.I.; Azmani, S.; Das, S.; Effendy, N. Consequences of Circadian Disruption in Shift Workers on Chrononutrition and their Psychosocial Well-Being. Int. J. Environ. Res. Public Health 2020, 17, 2043.
➤ Get "Smart Citations"
(hover to see more details)
.
Recent research highlights the interplay between diet, genetics, and gut microbiota in conditions like neurodegeneration, cancer, menopause symptoms, and Non-Alcoholic Fatty Liver Disease (NAFLD), all characterized by chronic inflammation ✅ Trusted Source
➤ Go to source
Gentile, F.; Doneddu, P.E.; Riva, N.; Nobile-Orazio, E.; Quattrini, A. Diet, Microbiota and Brain Health: Unraveling the Network Intersecting Metabolism and Neurodegeneration. Int. J. Mol. Sci. 2020, 21, 7471.
➤ Get "Smart Citations"
(hover to see more details)
✅ Trusted Source
➤ Go to source
Gill, S.K.; Rossi, M.; Bajka, B.; Whelan, K. Dietary fibre in gastrointestinal health and disease. Nat. Rev. Gastroenterol. Hepatol. 2021, 18, 101–116.
➤ Get "Smart Citations"
(hover to see more details)
✅ Trusted Source
➤ Go to source
Barnard, N.D.; Kahleova, H.; Holtz, D.N.; del Aguila, F.; Neola, M.; Crosby, L.M.; Holubkov, R. The Women’s Study for the Alleviation of Vasomotor Symptoms (WAVS): A randomized, controlled trial of a plant-based diet and whole soybeans for postmenopausal women. Menopause 2021, 28, 1150–1156.
➤ Get "Smart Citations"
(hover to see more details)
✅ Trusted Source
➤ Go to source
Zhao, H.; Yang, A.; Mao, L.; Quan, Y.; Cui, J.; Sun, Y. Association Between Dietary Fiber Intake and Non-alcoholic Fatty Liver Disease in Adults. Front. Nutr. 2020, 7, 593735.
➤ Get "Smart Citations"
(hover to see more details)
✅ Trusted Source
➤ Go to source
Nova, E.; Gómez-Martinez, S.; González-Soltero, R. The Influence of Dietary Factors on the Gut Microbiota. Microorganisms 2022, 10, 1368.
➤ Get "Smart Citations"
(hover to see more details)
.
Studies have shown that microbiota heritability exists in humans, particularly in twins ✅ Trusted Source
➤ Go to source
Goodrich, J.K.; Davenport, E.R.; Clark, A.G.; Ley, R.E. The Relationship Between the Human Genome and Microbiome Comes into View. Annu. Rev. Genet. 2017, 51, 413–433.
➤ Get "Smart Citations"
(hover to see more details)
✅ Trusted Source
➤ Go to source
Goodrich, J.K.; Davenport, E.R.; Beaumont, M.; Jackson, M.A.; Knight, R.; Ober, C.; Spector, T.D.; Bell, J.T.; Clark, A.G.; Ley, R.E. Genetic Determinants of the Gut Microbiome in UK Twins. Cell Host Microbe 2016, 19, 731–743.
➤ Get "Smart Citations"
(hover to see more details)
.
This suggests that the host can benefit from the intricate interactions between their genetic makeup and microbiome composition, influenced by factors like dietary patterns and environmental exposures ✅ Trusted Source
➤ Go to source
Goodrich, J.K.; Davenport, E.R.; Clark, A.G.; Ley, R.E. The Relationship Between the Human Genome and Microbiome Comes into View. Annu. Rev. Genet. 2017, 51, 413–433.
➤ Get "Smart Citations"
(hover to see more details)
.
However, there's a degree of variability in SCFA production among individuals.
For example, while acetate is typically the most abundant SCFA in feces, some individuals may exhibit substantial differences in propionate and butyrate levels.
This variability is an intriguing aspect of the SCFA story ✅ Trusted Source
➤ Go to source
Delgado, S.; Ruas-Madiedo, P.; Suárez, A.; Mayo, B. Interindividual Differences in Microbial Counts and Biochemical-Associated Variables in the Feces of Healthy Spanish Adults. Dig. Dis. Sci. 2006, 51, 737–743.
➤ Get "Smart Citations"
(hover to see more details)
.
How Our Bodies and Gut Bacteria Work Together
Host-Microbe Interface: Where It All Begins
Imagine your gut as a dynamic landscape.
The stomach's quick passage time and low acidity initially keep bacterial numbers low.
But as you progress into the small intestine, the scene changes ✅ Trusted Source
➤ Go to source
Donaldson, G.P.; Lee, S.M.; Mazmanian, S.K. Gut biogeography of the bacterial microbiota. Nat. Rev. Microbiol. 2016, 14, 20–32.
➤ Get "Smart Citations"
(hover to see more details)
✅ Trusted Source
➤ Go to source
Greenwood-Van Meerveld, B.; Johnson, A.C.; Grundy, D. Gastrointestinal Physiology and Function; Springer International Publishing: Berlin/Heidelberg, Germany, 2017; pp. 1–16.
➤ Get "Smart Citations"
(hover to see more details)
.
Bacterial populations increase substantially, setting the stage for the grand performance in the colon, where anaerobic bacteria thrive ✅ Trusted Source
➤ Go to source
Donaldson, G.P.; Lee, S.M.; Mazmanian, S.K. Gut biogeography of the bacterial microbiota. Nat. Rev. Microbiol. 2016, 14, 20–32.
➤ Get "Smart Citations"
(hover to see more details)
✅ Trusted Source
➤ Go to source
Pryde, S.E.; Duncan, S.H.; Hold, G.L.; Stewart, C.S.; Flint, H.J. The microbiology of butyrate formation in the human colon. FEMS Microbiol. Lett. 2002, 217, 133–139.
➤ Get "Smart Citations"
(hover to see more details)
.
One significant factor shaping this drama is pH levels ✅ Trusted Source
➤ Go to source
Fallingborg, J. Intraluminal pH of the human gastrointestinal tract. Dan. Med. Bull. 1999, 46, 183–196. .
The rise in pH from gastric acid neutralization and pancreatic secretions in the small intestine opens doors for specific bacteria.
The type of food you eat also matters, as it influences the fermentation products produced in your gut.
Diverse Diet, Diverse Microbiota
The availability of dietary fiber (DF) depends on what you eat.
DF serves as a substrate ✅ Trusted Source
➤ Go to source
Luca, S.V.; Macovei, I.; Bujor, A.; Miron, A.; Skalicka-Woźniak, K.; Aprotosoaie, A.C.; Trifa, A. Bioactivity of dietary polyphenols: The role of metabolites. Food Sci. Nutr. 2020, 60, 626–659.
➤ Get "Smart Citations"
(hover to see more details)
✅ Trusted Source
➤ Go to source
Recio, C.; Lucy, D.; Iveson, P.; Iqbal, A.J.; Valaris, S.; Wynne, G.; Russell, A.J.; Choudhury, R.P.; O’Callaghan, C.; Monaco, C.; et al. The Role of Metabolite-Sensing G Protein-Coupled Receptors in Inflammation and Metabolic Disease. Antioxid. Redox Signal. 2018, 29, 237–256.
➤ Get "Smart Citations"
(hover to see more details)
, and its composition influences the fermentation products generated.
In the colon, DF promotes the growth of diverse microbial species that engage in "substrate cross-feeding" ✅ Trusted Source
➤ Go to source
Flint, H.J.; Duncan, S.H.; Scott, K.P.; Louis, P. Interactions and competition within the microbial community of the human colon: Links between diet and health. Environ. Microbiol. 2007, 9, 1101–1111.
➤ Get "Smart Citations"
(hover to see more details)
✅ Trusted Source
➤ Go to source
Belenguer, A.; Duncan, S.H.; Calder, A.G.; Holtrop, G.; Louis, P.; Lobley, G.E.; Flint, H.J. Two Routes of Metabolic Cross-Feeding between Bifidobacterium adolescentis and Butyrate-Producing Anaerobes from the Human Gut. Appl. Environ. Microbiol. 2006, 72, 3593–3599.
➤ Get "Smart Citations"
(hover to see more details)
and "metabolic cross-feeding" ✅ Trusted Source
➤ Go to source
Heinken, A.; Thiele, I. Anoxic Conditions Promote Species-Specific Mutualism between Gut Microbes In Silico. Appl. Environ. Microbiol. 2015, 81, 4049–4061.
➤ Get "Smart Citations"
(hover to see more details)
.
These interactions enhance microbial diversity and create a harmonious environment in your colon.
One remarkable collaboration is between Faecalibacterium prausnitzii (F. prausnitzii) and Bacteroides thetaiotaomicron (B. thetaiotaomicron).
F. prausnitzii derives butyrate from acetate produced by B. thetaiotaomicron ✅ Trusted Source
➤ Go to source
Wrzosek, L.; Miquel, S.; Noordine, M.-L.; Bouet, S.; Chevalier-Curt, M.; Robert, V.; Philippe, C.; Bridonneau, C.; Cherbuy, C.; Robbe-Masselot, C.; et al. Bacteroides thetaiotaomicron and Faecalibacterium prausnitzii influence the production of mucus glycans and the development of goblet cells in the colonic epithelium of a gnotobiotic model rodent. BMC Biol. 2013, 11, 61.
➤ Get "Smart Citations"
(hover to see more details)
.
Butyrate, in turn, boosts intestinal barrier function and mucin production, fortifying your gut's defenses ✅ Trusted Source
➤ Go to source
Jung, T.-H.; Park, J.H.; Jeon, W.-M.; Han, K.-S. Butyrate modulates bacterial adherence on LS174T human colorectal cells by stimulating mucin secretion and MAPK signaling pathway. Nutr. Res. Pract. 2015, 9, 343.
➤ Get "Smart Citations"
(hover to see more details)
✅ Trusted Source
➤ Go to source
Peng, L.; Li, Z.-R.; Green, R.S.; Holzman, I.R.; Lin, J. Butyrate Enhances the Intestinal Barrier by Facilitating Tight Junction Assembly via Activation of AMP-Activated Protein Kinase in Caco-2 Cell Monolayers. J. Nutr. 2009, 139, 1619–1625.
➤ Get "Smart Citations"
(hover to see more details)
.
SCFA: Heroes of the Proximal Colon
Short Chain Fatty Acids (SCFA) are the stars of the show in the proximal colon ✅ Trusted Source
➤ Go to source
Topping, D.L.; Clifton, P.M. Short-Chain Fatty Acids and Human Colonic Function: Roles of Resistant Starch and Nonstarch Polysaccharides. Physiol. Rev. 2001, 81, 1031–1064.
➤ Get "Smart Citations"
(hover to see more details)
.
Acetate, propionate, and butyrate are the primary SCFA, absorbed by colonocytes ✅ Trusted Source
➤ Go to source
Ichikawa, H.; Sakata, T. Effect of L-Lactic Acid, Short-Chain Fatty Acids, and pH in Cecal Infusate on Morphometric and Cell Kinetic Parameters of Rat Cecum. Dig. Dis. Sci. 1997, 42, 1598–1610.
➤ Get "Smart Citations"
(hover to see more details)
and entering the bloodstream.
Their absorption and impact on crypt proliferation depend on luminal pH levels.
Butyrate, in particular, is a key player.
It serves as a major energy source for colonocytes, influencing their proliferation, differentiation, and mucus production ✅ Trusted Source
➤ Go to source
Pryde, S.E.; Duncan, S.H.; Hold, G.L.; Stewart, C.S.; Flint, H.J. The microbiology of butyrate formation in the human colon. FEMS Microbiol. Lett. 2002, 217, 133–139.
➤ Get "Smart Citations"
(hover to see more details)
.
Butyrate's protective role against colorectal carcinoma is well-documented, making it a vital component in gut health ✅ Trusted Source
➤ Go to source
Gill, P.A.; Zelm, M.C.V.; Muir, J.G.; Gibson, P.R. Review article: Short chain fatty acids as potential therapeutic agents in human gastrointestinal and inflammatory disorders. Aliment. Pharmacol. Ther. 2018, 48, 15–34.
➤ Get "Smart Citations"
(hover to see more details)
✅ Trusted Source
➤ Go to source
Frugé, A.D.; Smith, K.S.; Riviere, A.J.; Tenpenny-Chigas, R.; Demark-Wahnefried, W.; Arthur, A.E.; Murrah, W.M.; van der Pol, W.J.; Jasper, S.L.; Morrow, C.D.; et al. A Dietary Intervention High in Green Leafy Vegetables Reduces Oxidative DNA Damage in Adults at Increased Risk of Colorectal Cancer: Biological Outcomes of the Randomized Controlled Meat and Three Greens (M3G) Feasibility Trial. Nutrients 2021, 13, 1220.
➤ Get "Smart Citations"
(hover to see more details)
.
Digestive Enzymes
Our genes also influence our gut microbiota.
For instance, the AMY1 gene, which encodes salivary α-amylase, affects the oral and gut microbiota composition ✅ Trusted Source
➤ Go to source
Poole, A.C.; Goodrich, J.K.; Youngblut, N.D.; Luque, G.G.; Ruaud, A.; Sutter, J.L.; Waters, J.L.; Shi, Q.; El-Hadidi, M.; Johnson, L.M.; et al. Human Salivary Amylase Gene Copy Number Impacts Oral and Gut Microbiomes. Cell Host Microbe 2019, 25, 553–564.e557.
➤ Get "Smart Citations"
(hover to see more details)
.
Individuals with low AMY1 copy numbers digest carbohydrates differently from those with high copy numbers, impacting their microbiomes and SCFA production.
Variants in the LCT gene ✅ Trusted Source
➤ Go to source
Blekhman, R.; Goodrich, J.K.; Huang, K.; Sun, Q.; Bukowski, R.; Bell, J.T.; Spector, T.D.; Keinan, A.; Ley, R.E.; Gevers, D.; et al. Host genetic variation impacts microbiome composition across human body sites. Genome Biol. 2015, 16, 191.
➤ Get "Smart Citations"
(hover to see more details)
, responsible for lactase production, are correlated with Bifidobacterium abundance in the gut.
Lactase persistence can affect dairy consumption and medication responses, making genetics an essential factor in our gut's story.
Genetic Diversity and Barriers
The gut microbiota has a role in regulating our physical barriers, such as mucin production ✅ Trusted Source
➤ Go to source
Wrzosek, L.; Miquel, S.; Noordine, M.-L.; Bouet, S.; Chevalier-Curt, M.; Robert, V.; Philippe, C.; Bridonneau, C.; Cherbuy, C.; Robbe-Masselot, C.; et al. Bacteroides thetaiotaomicron and Faecalibacterium prausnitzii influence the production of mucus glycans and the development of goblet cells in the colonic epithelium of a gnotobiotic model rodent. BMC Biol. 2013, 11, 61.
➤ Get "Smart Citations"
(hover to see more details)
.
Mucin is a vital component of the mucus layer separating enterocytes and microbiota.
Polymorphisms in mucin genes are associated with gastric cancer risk, emphasizing the interplay between genetics and gut health ✅ Trusted Source
➤ Go to source
Wen, R.; Gao, F.; Zhou, C.-J.; Jia, Y.-B. Polymorphisms in mucin genes in the development of gastric cancer. World J. Gastrointest. Oncol. 2015, 7, 328.
➤ Get "Smart Citations"
(hover to see more details)
.
Maintaining Barrier Integrity
Short Chain Fatty Acids (SCFA) play a crucial role in maintaining the integrity of our gut barriers.
They upregulate tight junction proteins ✅ Trusted Source
➤ Go to source
Silva, Y.P.; Bernardi, A.; Frozza, R.L. The Role of Short-Chain Fatty Acids from Gut Microbiota in Gut-Brain Communication. Front. Endocrinol. 2020, 11, 25.
➤ Get "Smart Citations"
(hover to see more details)
, ensuring the gut remains impermeable.
This is especially important in preventing pathogen invasion, inflammation, and diseases like obesity and insulin resistance ✅ Trusted Source
➤ Go to source
Cani, P.D.; Bibiloni, R.; Knauf, C.; Waget, A.; Neyrinck, A.M.; Delzenne, N.M.; Burcelin, R. Changes in Gut Microbiota Control Metabolic Endotoxemia-Induced Inflammation in High-Fat Diet–Induced Obesity and Diabetes in Mice. Diabetes 2008, 57, 1470–1481.
➤ Get "Smart Citations"
(hover to see more details)
.
Immune Responses and Gut Health
Butyrate influences immune cell populations in the gut.
It promotes the differentiation of intestinal macrophages into tolerant macrophages, reducing pro-inflammatory responses ✅ Trusted Source
➤ Go to source
Chang, P.V.; Hao, L.; Offermanns, S.; Medzhitov, R. The microbial metabolite butyrate regulates intestinal macrophage function via histone deacetylase inhibition. Proc. Natl. Acad. Sci. USA 2014, 111, 2247–2252.
➤ Get "Smart Citations"
(hover to see more details)
.
Natural killer (NK) cell differentiation ✅ Trusted Source
➤ Go to source
Sanos, S.L.; Bui, V.L.; Mortha, A.; Oberle, K.; Heners, C.; Johner, C.; Diefenbach, A. RORγt and commensal microflora are required for the differentiation of mucosal interleukin 22–producing NKp46+ cells. Nat. Immunol. 2009, 10, 83–91.
➤ Get "Smart Citations"
(hover to see more details)
, essential for an impermeable intestinal barrier ✅ Trusted Source
➤ Go to source
Zheng, Y.; Valdez, P.A.; Danilenko, D.M.; Hu, Y.; Sa, S.M.; Gong, Q.; Abbas, A.R.; Modrusan, Z.; Ghilardi, N.; de Sauvage, F.J.; et al. Interleukin-22 mediates early host defense against attaching and effacing bacterial pathogens. Nat. Med. 2008, 14, 282–289.
➤ Get "Smart Citations"
(hover to see more details)
, is also influenced by SCFA ✅ Trusted Source
➤ Go to source
Zimmerman, M.A.; Singh, N.; Martin, P.M.; Thangaraju, M.; Ganapathy, V.; Waller, J.L.; Shi, H.; Robertson, K.D.; Munn, D.H.; Liu, K. Butyrate suppresses colonic inflammation through HDAC1-dependent Fas upregulation and Fas-mediated apoptosis of T cells. Am. J. Physiol.-Gastrointest. Liver Physiol. 2012, 302, G1405–G1415.
➤ Get "Smart Citations"
(hover to see more details)
✅ Trusted Source
➤ Go to source
Usami, M.; Kishimoto, K.; Ohata, A.; Miyoshi, M.; Aoyama, M.; Fueda, Y.; Kotani, J. Butyrate and trichostatin A attenuate nuclear factor κB activation and tumor necrosis factor α secretion and increase prostaglandin E2 secretion in human peripheral blood mononuclear cells. Nutr. Res. 2008, 28, 321–328.
➤ Get "Smart Citations"
(hover to see more details)
✅ Trusted Source
➤ Go to source
Park, J.; Kim, M.; Kang, S.G.; Jannasch, A.H.; Cooper, B.; Patterson, J.; Kim, C.H. Short-chain fatty acids induce both effector and regulatory T cells by suppression of histone deacetylases and regulation of the mTOR–S6K pathway. Mucosal Immunol. 2015, 8, 80–93.
➤ Get "Smart Citations"
(hover to see more details)
].
Antibiotic exposure in early life ✅ Trusted Source
➤ Go to source
Risnes, K.R.; Belanger, K.; Murk, W.; Bracken, M.B. Antibiotic Exposure by 6 Months and Asthma and Allergy at 6 Years: Findings in a Cohort of 1,401 US Children. Am. J. Epidemiol. 2011, 173, 310–318.
➤ Get "Smart Citations"
(hover to see more details)
can predict the risk of conditions like asthma.
Conversely, early exposure to diverse microorganisms may reduce the risk of asthma, allergies, and other disorders ✅ Trusted Source
➤ Go to source
Riaz Rajoka, M.S.; Thirumdas, R.; Mehwish, H.M.; Umair, M.; Khurshid, M.; Hayat, H.F.; Phimolsiripol, Y.; Pallarés, N.; Martí-Quijal, F.J.; Barba, F.J. Role of Food Antioxidants in Modulating Gut Microbial Communities: Novel Understandings in Intestinal Oxidative Stress Damage and Their Impact on Host Health. Antioxidants 2021, 10, 1563.
➤ Get "Smart Citations"
(hover to see more details)
✅ Trusted Source
➤ Go to source
Ege, M.J. The Hygiene Hypothesis in the Age of the Microbiome. Ann. Am. Thorac. Soc. 2017, 14, S348–S353.
➤ Get "Smart Citations"
(hover to see more details)
.
Prebiotics, such as GOS, increase beneficial bacteria, boost immune responses, and reduce inflammation ✅ Trusted Source
➤ Go to source
Vulevic, J.; Drakoularakou, A.; Yaqoob, P.; Tzortzis, G.; Gibson, G.R. Modulation of the fecal microflora profile and immune function by a novel trans-galactooligosaccharide mixture (B-GOS) in healthy elderly volunteers. Am. J. Clin. Nutr. 2008, 88, 1438–1446.
➤ Get "Smart Citations"
(hover to see more details)
.
Transporter Genetics: The SCFA Highway
Monocarboxylate transporters (MCTs) ✅ Trusted Source
➤ Go to source
Vulevic, J.; Drakoularakou, A.; Yaqoob, P.; Tzortzis, G.; Gibson, G.R. Modulation of the fecal microflora profile and immune function by a novel trans-galactooligosaccharide mixture (B-GOS) in healthy elderly volunteers. Am. J. Clin. Nutr. 2008, 88, 1438–1446.
➤ Get "Smart Citations"
(hover to see more details)
✅ Trusted Source
➤ Go to source
Vijay, N.; Morris, M. Role of Monocarboxylate Transporters in Drug Delivery to the Brain. Curr. Pharm. Des. 2014, 20, 1487–1498.
➤ Get "Smart Citations"
(hover to see more details)
are the highway for SCFA uptake by colonocytes.
Fourteen transporters, including MCT1 and MCT4, are responsible for SCFA transportation.
These transporters are influenced by genetic variations and play crucial roles in energy supply to different tissues.
MCTs are pleiotropic, meaning they have various downstream effects in different tissues ✅ Trusted Source
➤ Go to source
Felmlee, M.A.; Jones, R.S.; Rodriguez-Cruz, V.; Follman, K.E.; Morris, M.E. Monocarboxylate Transporters (SLC16): Function, Regulation, and Role in Health and Disease. Pharmacol. Rev. 2020, 72, 466–485.
➤ Get "Smart Citations"
(hover to see more details)
✅ Trusted Source
➤ Go to source
Schutkowski, A.; Wege, N.; Stangl, G.I.; König, B. Tissue-Specific Expression of Monocarboxylate Transporters during Fasting in Mice. PLoS ONE 2014, 9, e112118.
➤ Get "Smart Citations"
(hover to see more details)
.
For example, MCT2 is upregulated in neurons following food deprivation and ischemia recovery ✅ Trusted Source
➤ Go to source
Schutkowski, A.; Wege, N.; Stangl, G.I.; König, B. Tissue-Specific Expression of Monocarboxylate Transporters during Fasting in Mice. PLoS ONE 2014, 9, e112118.
➤ Get "Smart Citations"
(hover to see more details)
, while MCT4 is reduced in response to fasting ✅ Trusted Source
➤ Go to source
Rodriguez, J.E.R.; Garcia-Perdomo, H.A. Role of monocarboxylate transporters in the diagnosis, progression, prognosis, and treatment of prostate cancer. Turk. J. Urol. 2020, 46, 413–418.
➤ Get "Smart Citations"
(hover to see more details)
✅ Trusted Source
➤ Go to source
Ullah, M.S.; Davies, A.J.; Halestrap, A.P. The plasma membrane lactate transporter MCT4, but not MCT1, is up-regulated by hypoxia through a HIF-1alpha-dependent mechanism. J. Biol. Chem. 2006, 281, 9030–9037.
➤ Get "Smart Citations"
(hover to see more details)
.
These transporters also impact drug delivery, making them essential in therapeutic strategies.
SCFA Metabolism
SCFA are known to contribute significantly to human energy needs, comprising approximately 10% of our energy requirements ✅ Trusted Source
➤ Go to source
Van der Hee, B.; Wells, J.M. Microbial Regulation of Host Physiology by Short-chain Fatty Acids. Trends Microbiol. 2021, 29, 700–712.
➤ Get "Smart Citations"
(hover to see more details)
.
Among the various SCFA, butyrate stands out as the primary energy source for colonocytes, while propionate can be partially converted to glucose in the liver ✅ Trusted Source
➤ Go to source
Boets, E.; Gomand, S.V.; Deroover, L.; Preston, T.; Vermeulen, K.; de Preter, V.; Hamer, H.M.; van den Mooter, G.; de Vuyst, L.; Courtin, C.M.; et al. Systemic availability and metabolism of colonic-derived short-chain fatty acids in healthy subjects: A stable isotope study. J. Physiol. 2017, 595, 541–555.
➤ Get "Smart Citations"
(hover to see more details)
✅ Trusted Source
➤ Go to source
Bergman, E.N. Energy contributions of volatile fatty acids from the gastrointestinal tract in various species. Physiol. Rev. 1990, 70, 567–590.
➤ Get "Smart Citations"
(hover to see more details)
.
The GM plays a pivotal role in producing SCFA, generating an estimated 500–600 mmol/d of SCFA daily.
Of this, approximately 60% is acetate, 20% is propionate, and the remaining 20% is butyrate ✅ Trusted Source
➤ Go to source
Van der Hee, B.; Wells, J.M. Microbial Regulation of Host Physiology by Short-chain Fatty Acids. Trends Microbiol. 2021, 29, 700–712.
➤ Get "Smart Citations"
(hover to see more details)
.
These numbers translate to around 37 mmol/kg body weight of acetate, 13 mmol/kg body weight of propionate, and 12.4 mmol/kg body weight of butyrate ✅ Trusted Source
➤ Go to source
Van der Hee, B.; Wells, J.M. Microbial Regulation of Host Physiology by Short-chain Fatty Acids. Trends Microbiol. 2021, 29, 700–712.
➤ Get "Smart Citations"
(hover to see more details)
.
In the human descending colon, SCFA concentration can reach 69–91 mmol/kg luminal content, with acetate accounting for 60–75% of total fecal SCFA.
One notable player in this complex interplay is Methanobrevibacter smithii, found in 70% of humans, and a significant methane producer in the GM.
Studies suggest that methane production is linked to higher BMI scores in obesity, as well as conditions like constipation and antidepressant use ✅ Trusted Source
➤ Go to source
Basseri, R.J.; Basseri, B.; Pimentel, M.; Chong, K.; Youdim, A.; Low, K.; Hwang, L.; Soffer, E.; Chang, C.; Mathur, R. Intestinal methane production in obese individuals is associated with a higher body mass index. Gastroenterol. Hepatol. 2012, 8, 22–28. .
Additionally, in the presence of methane, propionate can be elongated to produce valerate ✅ Trusted Source
➤ Go to source
De Smit, S.M.; de Leeuw, K.D.; Buisman, C.J.N.; Strik, D.P.B.T.B. Continuous n-valerate formation from propionate and methanol in an anaerobic chain elongation open-culture bioreactor. Biotechnol. Biofuels 2019, 12, 132.
➤ Get "Smart Citations"
(hover to see more details)
.
Although less is known about valerate and caproate, they exist in small amounts in the gut and may impact health over time by altering the balance of acetate, butyrate, and propionate reaching human cells ✅ Trusted Source
➤ Go to source
Zhu, X.; Zhou, Y.; Wang, Y.; Wu, T.; Li, X.; Li, D.; Tao, Y. Production of high-concentration n-caproic acid from lactate through fermentation using a newly isolated Ruminococcaceae bacterium CPB6. Biotechnol. Biofuels 2017, 10, 102.
➤ Get "Smart Citations"
(hover to see more details)
.
Colon: A Hub of SCFA Activity
The colon plays a pivotal role in SCFA utilization, with more than 95% of the SCFA produced by the GM being absorbed here.
Butyrate, in particular, takes the spotlight as it accounts for over 70% of colonocyte energy production ✅ Trusted Source
➤ Go to source
Donohoe, D.; Garge, N.; Zhang, X.; Sun, W.; O’Connel, T.; Bunger, M.; Bultman, S. The Microbiome and Butyrate Regulate Energy Metabolism and Autophagy in the Mammalian Colon. Cell Metab. 2011, 13, 517–526.
➤ Get "Smart Citations"
(hover to see more details)
✅ Trusted Source
➤ Go to source
Donohoe, D.R.; Collins, L.B.; Wali, A.; Bigler, R.; Sun, W.; Bultman, S.J. The Warburg effect dictates the mechanism of butyrate-mediated histone acetylation and cell proliferation. Mol. Cell 2012, 48, 612–626.
➤ Get "Smart Citations"
(hover to see more details)
✅ Trusted Source
➤ Go to source
Jørgensen, J.; Mortensen, P.B. Utilization of short-chain fatty acids by colonic mucosal tissue strips. A new method of assessing colonic mucosal metabolism. Scand. J. Gastroenterol. 2000, 35, 659–666.
➤ Get "Smart Citations"
(hover to see more details)
.
Butyrate and propionate have been observed to increase cellular proliferation rates, while acetate does not share this effect ✅ Trusted Source
➤ Go to source
Cummings, J.H.; Gibson, G.R.; Macfarlane, G.T. Quantitative estimates of fermentation in the hind gut of man. Acta Vet. Scand. Suppl. 1989, 86, 76–82. ✅ Trusted Source
➤ Go to source
Den Besten, G.; van Eunen, K.; Groen, A.K.; Venema, K.; Reijngoud, D.-J.; Bakker, B.M. The role of short-chain fatty acids in the interplay between diet, gut microbiota, and host energy metabolism. J. Lipid Res. 2013, 54, 2325–2340.
➤ Get "Smart Citations"
(hover to see more details)
✅ Trusted Source
➤ Go to source
Freeman, H.J. Effects of differing concentrations of sodium butyrate on 1,2-dimethylhydrazine-induced rat intestinal neoplasia. Gastroenterology 1986, 91, 596–602.
➤ Get "Smart Citations"
(hover to see more details)
✅ Trusted Source
➤ Go to source
McIntyre, A.; Gibson, P.R.; Young, G.P. Butyrate production from dietary fibre and protection against large bowel cancer in a rat model. Gut 1993, 34, 386–391.
➤ Get "Smart Citations"
(hover to see more details)
.
Butyrate's significance extends to enterocyte differentiation, primarily driven by factors like FoxO3 and hypoxia-inducible factor α (HIF-α).
FoxO3 is associated with cellular homeostasis and longevity, suggesting a role for SCFA in epigenetic modulation and potential links to cancer development and tissue healing ✅ Trusted Source
➤ Go to source
Morris, B.J.; Willcox, D.C.; Donlon, T.A.; Willcox, B.J. FOXO3: A Major Gene for Human Longevity—A Mini-Review. Gerontology 2015, 61, 515–525.
➤ Get "Smart Citations"
(hover to see more details)
.
Butyrate also plays a role in inhibiting DNA-damaged cell proliferation via p53.
In intestinal crypts, a diffusion gradient allows butyrate to be metabolized by mature enterocytes, which, in turn, enhances intestinal permeability and immune function.
This process results in a reduction in intestinal permeability, an increase in TGB-β production promoting Treg differentiation, and a shift towards a more tolerogenic immune profile with increased levels of circulating IL-10 ✅ Trusted Source
➤ Go to source
Van der Hee, B.; Wells, J.M. Microbial Regulation of Host Physiology by Short-chain Fatty Acids. Trends Microbiol. 2021, 29, 700–712.
➤ Get "Smart Citations"
(hover to see more details)
.
Furthermore, butyrate may directly and indirectly improve intestinal permeability and immune function, potentially suppressing pro-inflammatory factors such as TNF-α and IL-6 ✅ Trusted Source
➤ Go to source
Liu, B.; Qian, J.; Wang, Q.; Wang, F.; Ma, Z.; Qiao, Y. Butyrate protects rat liver against total hepatic ischemia reperfusion injury with bowel congestion. PLoS ONE 2014, 9, e106184.
➤ Get "Smart Citations"
(hover to see more details)
.
Interestingly, studies have shown relatively indifferent usage of SCFA by colonic tissue in arterio-venous studies ✅ Trusted Source
➤ Go to source
Tsukahara, T.; Matsukawa, N.; Tomonaga, S.; Inoue, R.; Ushida, K.; Ochiai, K. High-sensitivity detection of short-chain fatty acids in porcine ileal, cecal, portal and abdominal blood by gas chromatography-mass spectrometry. Anim. Sci. J. 2014, 85, 494–498.
➤ Get "Smart Citations"
(hover to see more details)
.
Acetate, on the other hand, appears to be a potent stimulant of intestinal blood flow and plays a role in regulating the brain-pancreas axis regarding insulin release regulation ✅ Trusted Source
➤ Go to source
Perry, R.J.; Peng, L.; Barry, N.A.; Cline, G.W.; Zhang, D.; Cardone, R.L.; Petersen, K.F.; Kibbey, R.G.; Goodman, A.L.; Shulman, G.I. Acetate mediates a microbiome-brain-β cell axis promoting metabolic syndrome. Nature 2016, 7606, 213–217.
➤ Get "Smart Citations"
(hover to see more details)
.
Propionate, another SCFA, is gaining attention for its role in regulating colonic motility, and high doses of SCFA have been found to inhibit smooth muscle cell proliferation ✅ Trusted Source
➤ Go to source
Yuan, F.; Tan, W.; Ren, H.; Yan, L.; Wang, Y.; Luo, H. The Effects of Short-Chain Fatty Acids on Rat Colonic Hypermotility Induced by Water Avoidance Stress. Drug Des. Dev. Ther. 2020, 14, 4671–4684.
➤ Get "Smart Citations"
(hover to see more details)
✅ Trusted Source
➤ Go to source
Le Blay, G.; Blottière, H.M.; Ferrier, L.; le Foll, E.; Bonnet, C.; Galmiche, J.P.; Cherbut, C. Short-chain fatty acids induce cytoskeletal and extracellular protein modifications associated with modulation of proliferation on primary culture of rat intestinal smooth muscle cells. Dig. Dis. Sci. 2000, 45, 1623–1630.
➤ Get "Smart Citations"
(hover to see more details)
.
Genetic variations in MCT1 and MCT2, which are responsible for SCFA absorption in the gut, may affect colorectal cancer outcomes ✅ Trusted Source
➤ Go to source
Fei, F.; Guo, X.; Chen, Y.; Liu, X.; Tu, J.; Xing, J.; Chen, Z.; Ji, J.; He, X. Polymorphisms of monocarboxylate transporter genes are associated with clinical outcomes in patients with colorectal cancer. J. Cancer Res. Clin. Oncol. 2015, 141, 1095–1102.
➤ Get "Smart Citations"
(hover to see more details)
.
Additionally, ketone bodies produced from SCFA β-oxidation serve as precursors for lipid synthesis in human cells, with glucose and glutamine oxidation being more relevant for energy production in the small intestine and proximal colon ✅ Trusted Source
➤ Go to source
Macfarlane, G.T.; Macfarlane, S. Bacteria, Colonic Fermentation, and Gastrointestinal Health. J. AOAC Int. 2012, 95, 50–60.
➤ Get "Smart Citations"
(hover to see more details)
✅ Trusted Source
➤ Go to source
De Vadder, F.; Kovatcheva-Datchary, P.; Goncalves, D.; Vinera, J.; Zitoun, C.; Duchampt, A.; Bäckhed, F.; Mithieux, G. Microbiota-generated metabolites promote metabolic benefits via gut-brain neural circuits. Cell 2014, 156, 84–96.
➤ Get "Smart Citations"
(hover to see more details)
✅ Trusted Source
➤ Go to source
Soty, M.; Penhoat, A.; Amigo-Correig, M.; Vinera, J.; Sardella, A.; Vullin-Bouilloux, F.; Zitoun, C.; Houberdon, I.; Mithieux, G. A gut-brain neural circuit controlled by intestinal gluconeogenesis is crucial in metabolic health. Mol. Metab. 2015, 4, 106–117.
➤ Get "Smart Citations"
(hover to see more details)
.
Liver and Adipose Tissue: SCFA in Action
Studies in humans have revealed that butyrate ratios decrease from 20% in the gut lumen to 8% in portal blood ✅ Trusted Source
➤ Go to source
Cummings, J.H.; Gibson, G.R.; Macfarlane, G.T. Quantitative estimates of fermentation in the hind gut of man. Acta Vet. Scand. Suppl. 1989, 86, 76–82. .
SCFA are taken up by the portal circulation and used as energy substrates by hepatocytes, with propionate being a primary player in the liver ✅ Trusted Source
➤ Go to source
Morrison, D.J.; Preston, T. Formation of short chain fatty acids by the gut microbiota and their impact on human metabolism. Gut Microbes 2016, 7, 189–200.
➤ Get "Smart Citations"
(hover to see more details)
.
Specific transporters, MCT1 and MCT2, are predominantly expressed in cells using lactic acid for lipogenesis and gluconeogenesis, such as the liver, kidney tubules, and adipose tissue ✅ Trusted Source
➤ Go to source
Halestrap, A.P. The SLC16 gene family—Structure, role and regulation in health and disease. Mol. Asp. Med. 2013, 34, 337–349.
➤ Get "Smart Citations"
(hover to see more details)
.
Acetate is the only SCFA found in substantial amounts in the systemic circulation, reaching approximately 200 μM in venous serum ✅ Trusted Source
➤ Go to source
Van der Hee, B.; Wells, J.M. Microbial Regulation of Host Physiology by Short-chain Fatty Acids. Trends Microbiol. 2021, 29, 700–712.
➤ Get "Smart Citations"
(hover to see more details)
.
Arterial concentrations of acetate, propionate, and butyrate have been reported as 173, 3.6, and 7.5 mmol/l, respectively, for acetate, propionate, and butyrate, further underlining the hepatic clearance of SCFA ✅ Trusted Source
➤ Go to source
Bloemen, J.G.; Venema, K.; van de Poll, M.C.; Olde Damink, S.W.; Buurman, W.A.; Dejong, C.H. Short chain fatty acids exchange across the gut and liver in humans measured at surgery. Clin. Nutr. 2009, 28, 657–661.
➤ Get "Smart Citations"
(hover to see more details)
.
Research indicates that propionate plays a role in gluconeogenesis in the liver, while acetate and butyrate are utilized for fatty acid and cholesterol synthesis ✅ Trusted Source
➤ Go to source
Den Besten, G.; Lange, K.; Havinga, R.; van Dijk, T.H.; Gerding, A.; van Eunen, K.; Müller, M.; Groen, A.K.; Hooiveld, G.J.; Bakker, B.M.; et al. Gut-derived short-chain fatty acids are vividly assimilated into host carbohydrates and lipids. Am. J. Physiol. Gastrointest. Liver Physiol. 2013, 305, G900–G910.
➤ Get "Smart Citations"
(hover to see more details)
.
The impact of SCFA on the tricarboxylic acid (TCA) cycle and energy production varies depending on cell membrane receptors.
MCTs regulate the influx and efflux of SCFA, lactate, pyruvate, and ketone bodies, influencing nutrient access to different cell tissues ✅ Trusted Source
➤ Go to source
Halestrap, A.P. The SLC16 gene family—Structure, role and regulation in health and disease. Mol. Asp. Med. 2013, 34, 337–349.
➤ Get "Smart Citations"
(hover to see more details)
.
Studies have shown that MCT4, more abundant than MCT1 in glycolytic cells, regulates the transport of lactate from glycolyzing cells to respiratory cells, impacting cellular functions ✅ Trusted Source
➤ Go to source
Halestrap, A.P. The SLC16 gene family—Structure, role and regulation in health and disease. Mol. Asp. Med. 2013, 34, 337–349.
➤ Get "Smart Citations"
(hover to see more details)
.
Systemic Metabolism: A Complex Web of Effects
The effects of SCFA on the body are intricate, diverse, and sometimes indirect, with potential synergistic interactions.
Acetate and pyruvate, close relatives of SCFA, continually feed the TCA cycle, highlighting the ability to convert and reconvert between SCFA and the importance of acetate in energy production ✅ Trusted Source
➤ Go to source
Sakata, T. Pitfalls in short-chain fatty acid research: A methodological review. Anim. Sci. J. 2019, 90, 3–13.
➤ Get "Smart Citations"
(hover to see more details)
.
Propionate controls the TCA through its conversion to succinate, while butyrate, after β-oxidation, enters the TCA as acetate.
The impact of SCFA on the TCA and energy production is further regulated by cell membrane receptors, with MCTs playing a central role in the transport of SCFA, lactate, pyruvate, and ketone bodies ✅ Trusted Source
➤ Go to source
Blacher, E.; Levy, M.; Tatirovsky, E.; Elinav, E. Microbiome-Modulated Metabolites at the Interface of Host Immunity. J. Immunol. 2017, 198, 572–580.
➤ Get "Smart Citations"
(hover to see more details)
.
Recent research has shown that plasma levels of acetate, rather than fecal levels of SCFA, are linked to inflammatory markers and lipid subclasses in the blood, highlighting the importance of absorption ✅ Trusted Source
➤ Go to source
Kurilshikov, A.; van den Munckhof, I.C.L.; Chen, L.; Bonder, M.J.; Schraa, K.; Rutten, J.H.W.; Riksen, N.P.; de Graaf, J.; Oosting, M.; Sanna, S.; et al. Gut Microbial Associations to Plasma Metabolites Linked to Cardiovascular Phenotypes and Risk. Circ. Res. 2019, 124, 1808–1820.
➤ Get "Smart Citations"
(hover to see more details)
.
Another study suggests that circulating SCFA, particularly propionate, is associated with fasting GLP-1 levels and lipid metabolites, which could have implications for insulin sensitivity and metabolic health ✅ Trusted Source
➤ Go to source
Müller, M.; Hernández, M.A.G.; Goossens, G.H.; Reijnders, D.; Holst, J.J.; Jocken, J.W.E.; van Eijk, H.; Canfora, E.E.; Blaak, E.E. Circulating but not faecal short-chain fatty acids are related to insulin sensitivity, lipolysis and GLP-1 concentrations in humans. Sci. Rep. 2019, 9, 12515.
➤ Get "Smart Citations"
(hover to see more details)
.
The intricate interplay of SCFA in the body extends to the brain, where acetate, propionate, and butyrate can cross the blood-brain barrier, potentially influencing neurological functions ✅ Trusted Source
➤ Go to source
Perry, R.J.; Peng, L.; Barry, N.A.; Cline, G.W.; Zhang, D.; Cardone, R.L.; Petersen, K.F.; Kibbey, R.G.; Goodman, A.L.; Shulman, G.I. Acetate mediates a microbiome-brain-β cell axis promoting metabolic syndrome. Nature 2016, 7606, 213–217.
➤ Get "Smart Citations"
(hover to see more details)
.
Key Takeaways and Future Horizons in Gut Health Research
Current dietary fiber (DF) intake in Europe falls below recommended levels.
Increasing DF intake could reduce Non-Communicable Diseases (NCDs) by 15-30%.
SCFA, primarily produced from dietary fiber, has significant systemic effects.
They act as a crucial link between diet, gut microbiome, and health.
However, the causality of microbiota-derived metabolites in human disease remains unclear.
Research should prioritize human studies over in vitro findings.
The enterotype, which clusters gut bacterial communities, can have substantial health impacts.
SCFA effects are dose-dependent and vary among different types.
Colonocytes utilize SCFA for energy, influencing various metabolic pathways.
Future Perspectives:
Studies show that the gut microbiota can shift with dietary changes but remains resilient.
Precision dietary interventions, fecal microbiota transplantation, and synbiotics hold promise for addressing various diseases.
Environmental changes, like increased consumption of ultra-processed foods and decreased DF intake, may contribute to the rise in NCDs.
Complex interactions between the host, GM, and dietary patterns require further research, emphasizing the need for individualized approaches.
Investigating associations between dietary patterns, GM, and host genetics holds potential, but specific mechanisms behind phenotypic differences remain unknown.
Future research may pave the way for personalized therapies based on SCFA measurement, without disrupting overall wellbeing.
Review date not set.
How we reviewed this article:
Latest on:
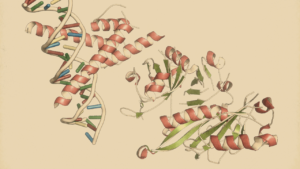