5 Key Benefits: Gut Fermentation of Dietary Fibers
Sections
Explore the transformative impact of gut fermentation of dietary fibers and its pivotal role in enhancing health and wellbeing. Delve into this comprehensive analysis now.
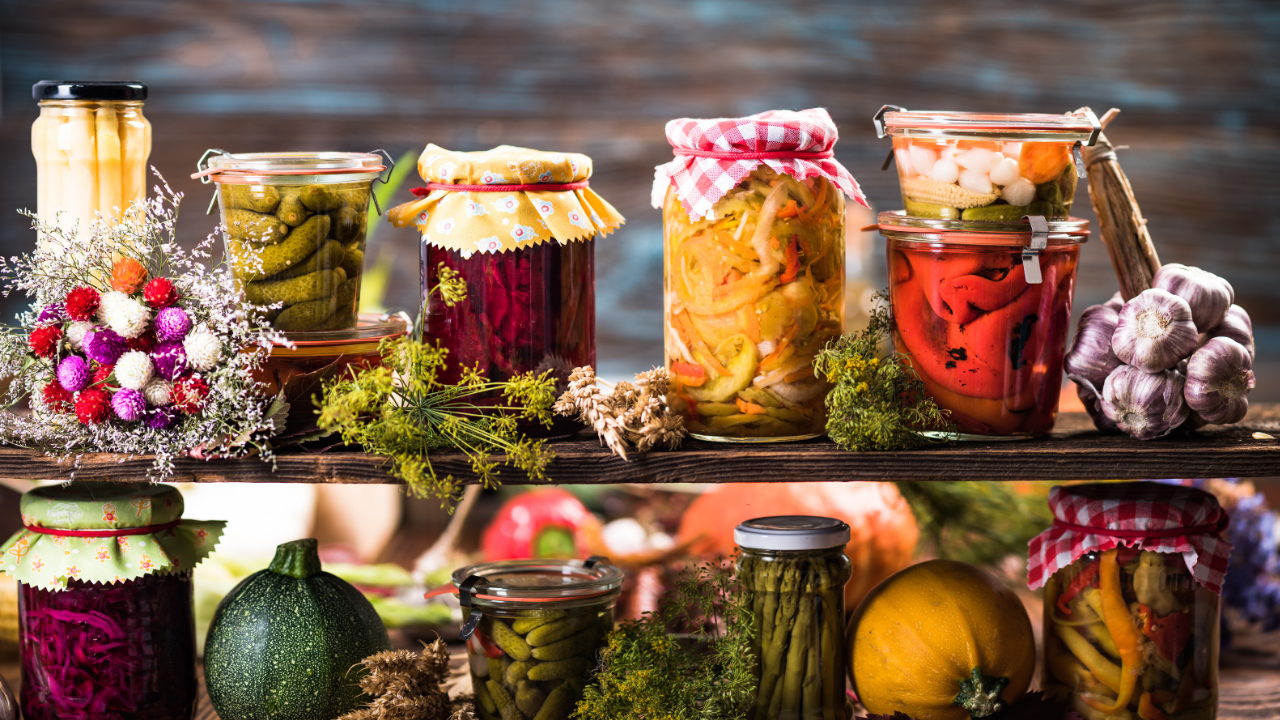
Review date not set.
How we reviewed this article:
Latest on:
Trending on:
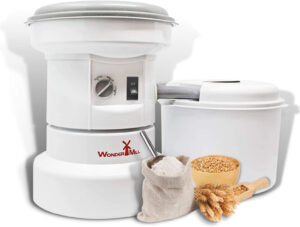
GrainMaster TurboChop Electric Mill
Experience lightning-fast milling with the TurboChop High Speed...
300 $
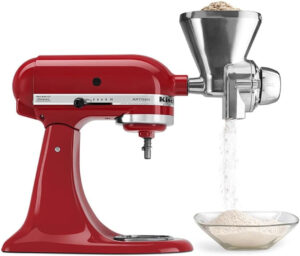
Grain Mill Mastery: KitchenAid KGM Attachment
Elevate your baking game with the KitchenAid KGM...
115 $
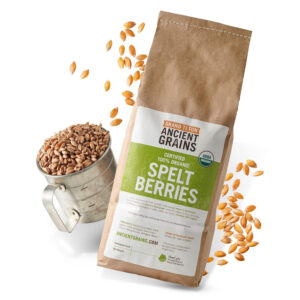
Nutrient-Packed Spelt Berries Supreme
Elevate your meals with our Supercharged Nutrient-Packed Spelt...
26 $